A Qualitative Assessment of the Risk of Acquiring Avian Influenza from Poultry and Game Bird Meat Poultry products
Avian influenza (AI) viruses cause infections primarily in bird species, although they are capable of spill-over infections into mammalian species, including humans. Many different strains of AI viruses are found in birds, but they can be divided into two groups based on their virulence in poultry: high pathogenicity (HPAI) and low pathogenicity (LPAI); both are capable of quickly spreading through a flock. HPAI infections often lead to severe clinical signs and high mortality while LPAI infections may not present with any clinical signs. Certain strains of AI have been associated with human case fatality rates of over 50%.
Since October 2021, there has been a substantial increase in the number of AI infections reported both at commercial premises and in wild birds in the UK. The last FSA assessment on the risk to consumers of exposure to AI from the food chain was in 2015. Since the increase in infections may lead to an increased likelihood that poultry products from infected birds are entering the retail market, an updated risk assessment was commissioned to ensure advice relating to the consumption of poultry products is still appropriate. This risk assessment did not focus on the currently circulating outbreak strain but considered any AI virus.
This assessment considered the risk of consumers acquiring an AI infection from poultry products, including commercial poultry, game birds, and table eggs. The risk of home processing of birds was also considered. The farm to fork risk pathway spanned from the probability that products from infected poultry would reach market to the ability of AI to cause infections in humans via the gastrointestinal route.
HPAI causes systemic infections in birds, so it can be found in feathers, blood, organs and muscle tissue. It may also be present on eggshells and in the yolk and albumen of eggs. For HPAI infections in commercial poultry, it was considered unlikely that meat and eggs from infected flocks would reach retail as the severity of clinical signs would lead to rapid detection of the infection and removal of the flock and any associated products from the food chain. Evidence also suggested that some game birds, like pheasants and grouse, infected with HPAI would unlikely be processed for consumption due to either their clinical signs preventing them from being available for shooting or trained hunters recognising signs of infection and thus disposing of those birds. However, some game birds, like wild ducks and geese, do not exhibit obvious clinical signs even when infected with HPAI viruses.
LPAI infections are mostly restricted to the respiratory and gastrointestinal tract in infected birds, although there is limited experimental evidence that these viruses can infect other tissues and contaminate eggs in some species of birds. The lack of clinical signs when birds are infected with LPAI means there is a greater probability infected birds may be processed to enter the food chain, but the virus is unlikely to be present in the products that would be reaching the consumer because little or no virus has been reported in muscle tissue or eggs.
Despite the increase in AI outbreaks around the world in the past two years, there has not been an observed increase in human infections. This suggests there is a sufficient species barrier for current circulating clades to limit humans becoming infected with AI. When human cases have occurred, infection usually follows close, direct contact with infected birds as opposed to consumption of poultry products. This, combined with the fact that AI viruses are heat-labile and inactivated by cooking, reduces the likelihood that infection would occur in humans even if exposed to poultry products contaminated with AI.
The risk of acquiring AI from poultry products was considered on a UK population basis; subpopulations were considered for some situations when evidence indicated the activity (consumption or processing) was undertaken infrequently. The likelihood of infection for people in the UK from handling and consuming commercial chicken or turkey is negligible (so rare that it does not merit to be considered) with low uncertainty. For the consumption of farmed duck and geese and wild game birds, the likelihood is very low (very rare but cannot be excluded) with medium uncertainty. Given the difference in likelihood for systemic infections between virus types, HPAI and LPAI were considered separately for home processing of birds. Since HPAI can be distributed in tissues throughout the bird and processing birds at home could potentially include exposure by inhalation, the likelihood of HPAI infection in people handling and home processing birds is low (rare but does occur). For LPAI, the likelihood is very low (very rare but cannot be excluded). Both of these situations are associated with medium uncertainty. The likelihood of infection for people in the UK from handling and consuming hen table eggs is very low (very rare but cannot be excluded) with low uncertainty. As for the severity of illness in humans from AI infection, this was considered high (severe illness: causing life-threatening or substantial sequelae or illness of long duration) with medium uncertainty. This reflects the high case fatality rate associate with AI infections in humans, even if mild infections are also possible.
Several key uncertainties remain after reviewing the available evidence. One is around the frequency with which poultry products from infected flocks may be reaching the UK market as the data is not available to estimate this. Since AI infections in humans can present in a variety of ways, there is the possibility that human cases and the associated transmission pathways are being missed in UK surveillance. A final uncertainty relates to the ability of AI to lead to infection in humans via consumption. There is little epidemiological evidence to support this transmission pathway, but there have also been few research studies investigating it.
Avian influenza (AI), also known as bird flu, causes infections in birds but can also infect other species, including humans. While a range of AI strains can infect birds, they can be grouped into two different types depending on the clinical signs they cause in poultry: high pathogenicity avian influenza (HPAI) and low pathogenicity avian influenza (LPAI). Both types of viruses can cause human illness if transmission occurs, with some strains of AI exhibiting human case fatality rates of over 50%.
Since October 2021, there has been a substantial increase in the number of AI infections reported in birds in the UK. The last FSA assessment on the risk of exposure to AI from the food chain was in 2015. Given the increased prevalence of AI in UK birds, the risk assessment was updated to make sure advice related to consumption of poultry products remains appropriate. This risk assessment did not focus on the currently circulating outbreak strain but considered any AI virus.
This risk assessment considered the risk of exposure in consumers to AI from poultry products, including commercial poultry, game birds, and table eggs. The risk from home processing of birds was also considered. The evidence came from published scientific literature, reports from other government departments and FSA research projects.
HPAI infections in birds often lead to severe clinical signs which would allow for detection of the illness before birds are slaughtered for consumption. LPAI infection may go unnoticed in birds, but the virus is unlikely to infect parts of the birds that will enter the food chain. In addition to this, there is very little evidence available that suggests AI can infect humans from consumption of AI-contaminated poultry products.
Risk of acquiring AI from handling and consuming poultry
The risk of acquiring AI from poultry products was considered on a UK population basis; subpopulations were considered for some situations when evidence indicated the activity (consumption or processing) was undertaken infrequently. The likelihood of infection for people in the UK from handling and consuming commercial chicken or turkey is negligible (so rare that it does not merit to be considered) with low uncertainty. For the consumption of farmed duck and geese and wild game birds, the likelihood is very low (very rare but cannot be excluded) with medium uncertainty.
Risk of acquiring AI from home processing birds
Given the difference in systemic infections between virus types, HPAI and LPAI were considered separately for home processing of birds. Since HPAI can be distributed in tissues throughout the bird and this pathway could potentially include exposure by inhalation, the likelihood of HPAI infection in people handling and home processing game birds is low (rare but does occur). For LPAI, the likelihood is very low (very rare but cannot be excluded). Both of these situations are associated with medium uncertainty.
Risk of acquiring AI from consuming hen table eggs
The likelihood of infection for people in the UK from handling and consuming hen table eggs is very low (very rare but cannot be excluded) with low uncertainty. As for the severity of illness in humans from AI infection, this was considered high (severe illness: causing life-threatening or substantial sequelae or illness of long duration) with medium uncertainty. This reflects the high case fatality rate associated with AI infections in humans, even if mild infections are also possible.
Locations around the world, including the UK, are currently experiencing a period of prolonged outbreaks of AI in commercial poultry and wild birds. The last FSA risk assessment produced on the risk to consumers of AI exposure via food was published in 2015; this risk assessment was reviewed and endorsed by the Advisory Committee on the Microbiological Safety of Food (ACMSF, 2015). This assessed that the risk from thoroughly cooked poultry products, including eggs, was very low. In 2017, the FSA changed its advice on eating less than thoroughly cooked (LTTC) eggs to indicate that eggs produced under the British Lion Code or equivalent schemes were safe to eat by most vulnerable groups (FSA, 2022a). Given this change in advice on egg consumption, the fact that some poultry meat, like duck, may be consumed pink, and the high number of AI infections in birds in the UK at the present time, this risk assessment was commissioned to determine the risk to UK consumers of acquiring AI from poultry products. This risk assessment did not focus on the currently circulating outbreak strain but considered any AI virus.
1.1 Risk Question
What is the risk to consumers, on a population basis, of becoming ill with AI viruses via consumer handling of feathered birds and food consumption, specifically of poultry, game meat, and eggs, during an AI season?
1.1.1 In scope
- any AI virus. This risk assessment is not focused solely on the HPAI H5N1 clade 2.3.4.4b circulating in the UK during the 2021/22 and 2022/23 AI seasons.
- meat from commercial poultry production (primarily chickens and turkeys, but includes some farmed duck and geese)
- exposure resulting from the preparation and consumption of wild game purchased in-feather by the consumer (obtained from Animal Game Handling Establishments or taken directly home)
- preparation and consumption of poultry products from backyard flocks
- table eggs from UK hen laying flocks
- food made at home using lightly cooked/raw UK hen eggs
- any of the above poultry products consumed less than thoroughly cooked
- cross-contamination from any of the above products
1.1.2 Out of scope
- animal health risks of acquiring AI
- occupational exposure from commercial poultry production
- exposure to birds outside the context of slaughtering, processing or consumption. The risk from general exposure to birds is considered by the UKHSA risk assessment (UKHSA, 2023).
- poultry products cooked by businesses to be consumed ready-to-eat
- eggs other than hen table eggs
- imported poultry products
1.1.3 Key Assumptions
In reviewing the evidence to determine the risk to humans of exposure to AI from poultry products, several key assumptions were made to assist in drawing conclusions when a lack of data was available. These included:
- uncharacterised strains of HPAI or LPAI will behave similarly to strains where clinical signs in birds, viral distribution in tissues, and viral survival have been studied.
- in bird species where AI infection has not been well characterised, that these birds would react to AI infection in a manner similar to well-studied related species (For example, same family or order) with regards to development of clinical signs and viral distribution within tissues.
- that AI viruses would behave similarly to viruses from previous AI outbreaks in their ability to mutate and/or transmit between species.
1.2 Background
Avian influenza (AI) is a disease in birds caused by AI viruses. AI viruses have occasionally infected other species, including humans. They are introduced into the UK via migratory birds, so outbreaks usually show a seasonal nature following migratory patterns. Table 1 provides details of previous outbreaks of AI in the UK for which APHA has produced epidemiology reports (Defra, 2021). Until September 2021, any cases detected in flocks in the UK were able to be relatively controlled through culling of infected premises, increased surveillance, and additional biosecurity measures. During this time, the disease also did not present over the summer months.
Table 1: Reported poultry outbreaks of AI in the UK. Data obtained from (Defra, 2021) and (Defra, 2023a). NR = none recorded
Avian Influenza season | HPAI | LPAI |
---|---|---|
October 2013 to September 2014 | NR | NR |
October 2014 to September 2015 |
1 IP* - H5N8 1 IP - H7N7 |
1 IP - H7N7 |
October 2015 to September 2016 | NR | 1 IP - H5N1 |
October 2016 to September 2017 | 13 IP - H5N8 | NR |
October 2017 to September 2018 | NR | NR |
October 2018 to September 2019 | NR | NR |
October 2019 to September 2020 | NR | 1 IP - H5N3 |
October 2020 to September 2021 |
2 IP - H5N1 20 IP - H5N8 |
1 IP - H5N2 1 IP - H5N3 |
October 2021 to September 2022 | 152 IP - H5N1 | NR |
October 2022 to February 6th 2023 | 169 IP - H5NI | NR |
*IP = infected premises
1.2.1 Current avian influenza outbreak in the UK
The first detection of the currently circulating H5N1 strain in the UK was on October 15th, 2021. Unlike previous years, cases were recorded over summer months in the UK. By the end of the AI season on September 30th, 2022, 152 infected premises (IPs) had been confirmed in the UK. Infected premises can consist of commercial poultry farms or non-commercial operations, such as backyard flocks or zoos. Across England, Scotland and Wales, 1,727 wild birds tested positive for AI, spanning 410 unique locations (Freath et al., 2022).
The current October 2022 AI season has proven even more aggressive than the previous year. Based on Defra’s February 6th report, there were confirmed cases of H5N1 at 169 infected premises (131 commercial and 38 non-commercial). In that time, 794 wild birds had tested positive for H5N1, spanning 350 unique locations (Defra, 2023a). There has also been one detection of H6N2 at an infected premises in England (UKHSA, 2022), but further information on whether it was HP or LP was not provided so it is not included in Table 1.
1.3 Related legislation
1.3.1 Poultry
Poultry keepers with more than 50 birds in England, Scotland and Wales are required to be registered with British Poultry Register (GOV.UK, 2022a). Given this, data on commercial poultry will not account for production from backyard or smallholder community flocks. The size of the backyard population in total is unknown (uncertainty).
As outlined in the Avian Influenza and Influenza of Avian Origin in Mammals (England) (No.2) Order 2006, which came into effect on 1st July 2006, commercial poultry premises are defined as “premises where poultry or other captive birds are kept for commercial purposes” and non-commercial premises as “premises where poultry or other captive birds are kept by their owners for their own consumption or use or as pets.”
1.3.2 Avian Influenza
The Avian Influenza and Influenza of Avian Origin in Mammals (England) (No.2) Order 2006 lays down measures to reduce the risk of the transmission of AI by outlining how to deal with suspected outbreaks and provides for surveillance of the disease. Similar measures are provided by Orders in Scotland and Wales (The Avian Influenza and Influenza of Avian Origin in Mammals (Wales) (No 2) Order 2006 and The Avian Influenza and Influenza of Avian Origin in Mammals (Scotland) Order 2006). Much of the orders focus on how to prevent spread of the disease to other birds, although reference is also made to reduce the risk of spread to other species.
In response to the AI outbreak in the UK in 2022, an amendment was introduced to the above order (The Avian Influenza and Influenza of Avian Origin in Mammals (Amendment) (England) Order 2022), which states, “These measures firstly amend the requirement for the Secretary of State to destroy all poultry meat traced from infected premises to allow the Secretary of State the discretion to authorise the movement of this poultry meat to market; secondly the requirement to carry out a physical examination of poultry in protection and surveillance zones in the 24 hours prior to movement for slaughter is amended to allow remote visual inspections where authorised by a veterinary inspector.” This amendment authorises the movement of meat derived from the poultry originating from infected premises (produced in unregulated period) to wholesale or retail premises or for further processing where, following a risk assessment, the Secretary of State is satisfied that such movement does not endanger human or animal health. These measures will remain in effect until April 16th, 2023.
1.3.2.1 UK Biosecurity Measures introduced when avian influenza is detected
Where AI is suspected or confirmed, control zones may be put in place within which measures to reduce the risk of spread are imposed (Defra, 2022a). When highly pathogenic AI has been confirmed in poultry or any other captive birds, the following are put in place around the infected premises:
In the 3km protection zone (PZ), the following biosecurity measures apply: poultry and other captive birds are housed indoors, disposal of any bird carcasses when instructed by a veterinary inspector, poultry litter, manure and slurry must not be spread or removed from the premises, poultry and other captive birds are not to be moved onto or off the premises without a licence, eggs and meat are not to be moved inside/outside a zone without the licence (Defra, 2022a). These measures are in addition to the measures followed for the 10km surveillance zone (SZ).
In the 10km SZ, the movement of all poultry and eggs that enter or leave premises must be recorded (except for table eggs that are being moved direct to wholesale or retail premises to be sold directly to the consumers). Poultry, other captive birds, or mammals (including pigs) are not to be moved to or from premises where poultry or other captive birds are being kept without license. Poultry litter, manure or slurry, is not to be spread or removed from the premises. (Defra, 2022a).
If an LPAI has been confirmed, in poultry or other captive birds, a 1km restricted zone (RZ) may be put in place (Defra, 2022a).
1.3.2.2 Changes to poultry processing during avian influenza outbreaks
Before receiving poultry from premises within AI PZs, SZs, or RZs, slaughterhouses need to be inspected and pre-designated by FSA to then be designated by Defra, Welsh Government, or Scottish Ministers, in order to process such poultry (FSA Manual for Official Controls). These designated slaughterhouses will belong to either Level 1 (receive and process poultry from premises within a SZ or RZ) or Level 2 (handling restricted meat from the poultry originating from a PZ) (FSA, 2022b).
Based on Defra advice from 2017 regarding rules on meat produced from poultry and farmed game birds originating in PZs, meat from poultry or farmed game birds can be moved or sold, subject to a number of conditions (Defra, 2017a). One requirement is the oval identification mark being replaced with a specific mark (approved by the Secretary of State for each outbreak) which must remain throughout any processing and repacking (Defra, 2017a). Food Business Operators (FBOs) receiving live poultry, live farmed game and farmed game carcasses from protected zones must ensure that they are cut, transported, and stored separately from other meat (Defra, 2017a). Some small throughput slaughterhouses are not eligible to apply a health mark or identification mark to meat as they are under approval of Local Authorities, not the FSA (Defra, 2017a).
Production of poultry meat, minced meat, meat products and meat preparations from PZs, SZs, or RZs, can take place under Commission Regulations 852/2004, 853/2004, and 2017/625 (OCR). Meat from poultry originating from a PZ, meat from poultry originating from an area that subsequently became a PZ and was slaughtered within 20 or fewer days of the date estimated by a veterinary inspector as being the earliest date of infection at a premises in the relevant zone or meat that has not been kept separate from the above becomes “restricted meat” and a special mark is applied (Defra, 2017a). Restricted poultry meat and products from healthy birds originating from a PZ can be marketed within the UK with no further treatment (FSA Manual for Official Controls). Where birds from a PZ are slaughtered and processed, this part of the slaughterhouse and equipment must be cleaned and disinfected before other poultry is slaughtered and processed. Failure in this process can result in cross-contamination. In addition, all biosecurity measures must be implemented, as a failure to adhere to these may result in the spread of avian influenza. Poultry from PZ premises that have arrived at slaughterhouses are under official control, and must be placed in lairage, slaughtered, chilled and stored separately from products that are not under official control, until it is wrapped and packed (FSA Manual for Official Controls). All premises, vehicles and equipment can be a potential source of contamination; for example, mud, slurry, animal faeces, excretions, feathers, or any other similar organic matter may be potential sources of AI.
Meat produced from poultry or farmed game birds originating within a protection zone can be moved or sold, subject to several conditions set out in General Licence EXD249(HPAI)(EV) (Bird flu (avian influenza) movement licences, 2022; EXD249(HPAI)(EW), 2022).
Designated slaughterhouses are required to notify their clients that the slaughterhouse has been receiving and processing poultry from premises within a PZ for avian influenza (FSA Manual for Official Controls). Bird meat originating from the Infected Premises (IP), slaughtered within 21 days of the date estimated as being the earliest date of infection must, be traced and detained, and Defra might decide to dispose of that meat (FSA Manual for Official Controls) or, until 16th April 2023, authorise in writing the movement of such meat to wholesale or retail premises or for further processing where, following a risk assessment, Defra is satisfied that such movement does not endanger human or animal health.
1.4 Previous risk assessments for avian influenza in poultry products
In 2006, the European Food Safety Authority (EFSA) Scientific Panel on Biological Hazards considered the evidence that food could serve as a source of infection with HPAI and concluded that “there was no epidemiological evidence to date that avian influenza can be transmitted to humans through consumption of food, notable poultry and eggs” (EFSA, 2006).
In 2010, the USDA’s Food Safety and Inspection Service (FSIS) published a quantitative risk assessment on the public health impact of HPAI virus in poultry, shell eggs and egg products. For poultry, the model determined that there was about a 5% probability that an infected chicken may be sent for slaughter undetected. However, this probability of exposure to AI from poultry meat was reduced to negligible if the poultry was cooked to the USDA FSIS recommended 165F (73.9 °C), as that temperature would inactivate the virus. Similarly, they predicted that some HPAI virus-contaminated eggs may be collected for release to market, but that the length of the distribution chain would allow for subsequent detection and removal of these eggs to reduce the risk to public health. Additionally, pasteurisation time and temperature combinations typically used for egg products would inactivate the virus, resulting in negligible risk to public health.
The risk to humans from transmission of low pathogenicity avian influenza in poultry meat and table eggs was considered by EFSA in 2018 (EFSA et al., 2018). They concluded that the probability of infection with LPAI virus from consuming commercial poultry and table eggs was negligible. The risk of infection from handling and/or manipulation of raw poultry meat was very unlikely. The report noted that there was a high level of uncertainty related to the different steps of the transmission pathways due to the limited number of research studies available to inform them (EFSA et al., 2018).
1.5 Current non-UK advice on avian influenza and poultry products
The WHO advice on whether or not it is safe to eat poultry products produced in areas experiencing an AI outbreak, last updated in 2020, says “meat products and eggs can be safely consumed, provided they are properly prepared because influenza viruses are inactivated by thorough cooking.” It recommends against eating raw or incompletely cooked meat and eggs from areas experiencing outbreaks (WHO, 2020).
The CDC is in agreement with the WHO and recommends that all poultry meat and egg products are properly cooked before eating as this will kill any AI virus. Although there is no evidence of human AI infection after eating properly cooked poultry products, they do recognise that uncooked poultry products, like blood, may have contributed to a small number of human infections in Asia (CDC, 2022a).
2.1 Avian Influenza viruses
Influenza A viruses are negative-sense, single-stranded RNA viruses classified in the family Orthomyxoviridae, with a size ranging from 80-120nm (Spackman, 2020). Domestic poultry are especially vulnerable due to the intensive nature of poultry farming. The virus can spread rapidly, causing outbreaks in commercial flocks.
2.1.1 Subtypes and pathogenicity
Type A influenza strains are classified by the serological subtypes of the primary viral surface proteins; the hemagglutinin (HA) and neuraminidase (NA) (Hutchinson, 2018). Sixteen HA subtypes and nine NA subtypes can be found in birds, which are the primary reservoir for the virus (CDC, 2022b). AI can be divided into high pathogenicity (HPAI) and low pathogenicity (LPAI) strains based on their ability to cause disease in poultry (Liu et al., 2021). Mutations from LPAI to HPAI are possible and have been described in Australia, the Netherlands, UK and Germany (Byrne et al., 2021). HPAI results in high death rates (up to 100% mortality within 48 hours) in some poultry species such as chickens and turkeys, while LPAI viruses may cause no disease or only mild clinical signs (such as ruffled feathers and a drop in egg production) and may go undetected (Liu et al., 2021).The vast majority of human cases have reported close contact with poultry; there is no reported evidence of sustained human-to-human transmission (“WHO, 2023,”). It’s worth noting that LPAI and HPAI refers only to the specific criteria in infected poultry, not the severity of illness with human infections (CDC, 2022c). The clinical illness associated with human infections with AI do not correlate with viral pathogenicity in infected birds.
The primary risk factor for human infection appears to be direct or indirect exposure to infected live or dead poultry or contaminated environments, such as live bird markets. Slaughtering, defeathering, handling carcasses of infected poultry, and preparing poultry for consumption, especially in household settings, are also likely to be risk factors. There is no evidence to suggest that AI viruses can be transmitted to humans through properly handled and cooked poultry or eggs. H5N1 human cases have been linked to consumption of dishes made with raw, contaminated poultry blood (WHO, 2018).
2.1.2 AI virus circulation in poultry populations in the UK in 2021/22 and 2022/23 seasons
The AI strain associated with the current outbreak belongs to the H5N1 clade 2.3.4.4b. Twelve different genotypes of H5N1 have been identified in commercial poultry and wild birds by WGS throughout this current outbreak (UKHSA, 2022).
The first genotype detected in the outbreak was AIV07, however divergence was subsequently detected, and it was reclassified into two separate genotypes: AIV07-B1 and AIV07-B2. The AIV07-B1 genotype contains a HA with high similarity to the H5N1 virus from 2020/21 and was the primary UK H5N1 genotype detection during 2021/22. However, it later became a minority population and has not been detected in the UK after February 2022. The AIV07-B2 genotype, possesses a HA gene that has diverged from AIV07-B1.
AIV09 is now the most prevalent genotype. It was initially detected in November 2021 and has subsequently been found across the UK in 297 poultry and wild birds. The PB2 segment of this genome has high similarity to H5N3 which circulated through Europe in 2020/21.
Genotypes such as AIV20, AIV55 and AIV08 have only been detected once in commercial farms in February 2022, December 2021, and October 2021 respectively. However, the PB2 segment of genotype AIV08 has high similarity to several LPAI detected in Europe since 2020.
2.1.2.1 Mammalian infections from current strains of HPAI H5N1
There have also been a number of cases of HPAI H5N1 identified in mammals. For example, H5N1 virus was detected in the brains of three red foxes in the Netherlands which presented with neurological signs (Vreman et al., 2022). The H5N1 virus which caused these infections was found to have mutation E627K in PB2. The PB2-E627K mutation has previously been found to increase virus replication, in vitro, in mammalian cell lines (Bordes et al., 2023) and pathogenicity, in vivo, in mice (Peng et al., 2018). This particular mutation is in the virus polymerase protein, and likely increases virus replication at the lower body temperature of mammals compared to birds (Steel et al., 2009). Another recent example of H5N1 mammalian infection was in a Mink farm in Spain in October 2022. Clinical signs of infection in the minks included neurological manifestations such as ataxia and tremors. The H5N1 virus isolated in this outbreak also had a mutation in PB2 at position 271.
In the UK, 14 animals have been found positive for AI H5N1 since 2021, including Eurasian Otters and Red Foxes (APHA, 2023). When whole genome sequences were obtained from these positive cases, the vast majority showed the PB2-E627K mutation. No other mammalian adaptive mutations have been detected so far in the country.
The dominant circulating H5N1 genotypes in avian species since October 2021, AIV09 and AIV07-B2, do not contain this mutation and despite these mutations for increased mammalian susceptibility appearing, no viruses have been detected with mutations associated to increased tropism of the human receptor for virus entry (Vreman et al., 2022).
Several epidemiological risk factors have been highlighted by Harris et al, as increasing the likelihood of transmission of AI between animal species (Harris et al., 2017). These include close contact with coastal birds or bird faeces (transmission from birds to marine mammals) and close proximity to farms (transmission between pigs and turkeys). The largest epidemiological risk factor for transmission from animal to humans was identified as direct, close and prolonged exposure to infected animals, particularly dead or sick poultry. Age also appears to be a factor in the likelihood of transmission of HPAI H5N1 to humans as infections have most commonly been seen in children and young adults, compared to HPAI H7N9 and H9N2. Despite the results of this paper there is still a significant knowledge gap relating to epidemiological factors associated with cross-species transmission (uncertainty).
2.2 Foods of concern for avian influenza contamination
Of all the documented cases of AI in humans, none have been attributed to the consumption of thoroughly cooked food, including eggs.
Epidemiological evidence suggests that most human infections with AI viruses have occurred following direct or close contact with infected (ill or dying) poultry (CDC, 2022d; Wiwanitkit, 2007).
Transmission of the H5N1 virus to mammals has been observed when domestic cats or tigers and leopards were fed with infected poultry (Keawcharoen et al., 2004; Kuiken et al., 2004). Some human infections are suspected to have occurred following the consumption of fresh duck blood; one news article reported a Vietnamese women tested positive for the H5N1 strain, following drinking duck blood (“Vietnamese Has Bird Flu After Drinking Duck Blood,” 2005). Given this evidence of transmission from poultry products to other mammalian species, including humans, this risk assessment considered the risk to humans of exposure to AI from a variety of poultry products.
3.1 Avian Influenza infections in humans
Although AI does not infect people easily and is not usually spread from person to person, there have been cases of infections in humans, with high mortality in some instances. The first laboratory-confirmed case in a human occurred in 1997, when H5N1 was detected in a patient in China (Wang et al., 2021). A majority of AI cases in humans come from the Western Pacific Region, including China, likely due to the widespread practice of keeping poultry in the backyard and the live poultry markets common in the region (Skufca et al., 2022). However, since 2003, AI has travelled out of the region and caused human infections all over the world (Wang et al., 2021).
AI H7N9 has caused the largest number of human infections worldwide, with 1,568 cases reported and 616 deaths, giving a 39% case-fatality rate; no human AI infections with an H7N9 strain have been reported since 2019 (EFSA, 2023; WHO, 2023). Following that, AI H5N1 has caused 868 cases worldwide in humans with 457 deaths, giving a 53% case fatality rate (WHO, 2022). AI H5N6 has also been associated with a high case fatality rate of 40% (83 cases and 33 deaths), although this strain has not been reported in humans outside of the Western Pacific Region (EFSA, 2023; WHO, 2023).
Other AI subtypes have been associated with human infections but either are not associated with a high case fatality rate or have caused a very small number of human infections worldwide. For example, AI H9N2 has been responsible for 115 human cases of AI but with only two reported fatalities. Between February and May 2003, an outbreak of H7N7 subtype in the Netherlands caused 89 human infections (Fouchier et al., 2004). H5N2 AI strain has caused 20 human cases in Japan in 2005 (De Nardi et al., 2014). H5N8 led to 7 human cases in Russia in 2021 (EFSA, 2023). A very small number of human cases (1-3 each) have been associated with the following AI strains: H3N8, H6N1, H7N2, H7N3, H7N4, H10N3, H10N7, and H10N8 (EFSA, 2023; Skufca et al., 2022; WHO, 2023).
In the current H5N1 outbreak, six infections in humans have been reported (WHO, 2022). Four of these have occurred in Europe and the USA; one of these was in the UK and is discussed below (UKHSA, 2022). These were all either asymptomatic or reported only fatigue (EFSA, 2023). One fatal human infection of H5N1 has been reported from China in October 2022 (EFSA, 2023).
In late February 2023, the death of a young girl in Cambodia from avian influenza was confirmed. Testing of close contacts of the girl revealed her father was also infected, who reported experiencing a fever and cough. Sequencing determined the girl was infected with H5N1 2.3.2.1c, which is not related to the strain causing the current outbreaks in poultry around the world. This strain has been circulating in poultry and last caused infections in humans in Cambodia in 2014. It is believed contact with infected poultry led to these infections and there is no evidence that human to human transmission was responsible for the two family members becoming infected (CDC, 2023; Mallapaty, 2023).
3.1.1 UK avian influenza cases in humans
Infections with LPAI strains have been reported several times in the UK. The first case of AI reported in the UK was an LPAI H7N7 case in 1996 that resulted in conjunctivitis (CDC, 2022d; Wang et al., 2021). Sporadic cases of H7N2 and H7N3 were recorded in the UK between 2002 and 2007, which resulted in conjunctivitis and mild upper respiratory tract symptoms (CDC, 2022d; Wang et al., 2021).
The first human case of HPAI H5N1 avian influenza in the UK was confirmed in December 2021 (Oliver et al., 2022). The case was asymptomatic and only detected as the UKHSA had increased its surveillance of potentially exposed human contacts given the dramatic increase in H5N1 infections in birds in the UK. The individual was tested after a confirmed outbreak in their domestic flock of Muscovy ducks. The epidemiological investigation suggested that close contact with the flock and their contaminated environment was the likely source of infection (Oliver et al., 2022).
The UKHSA are working together with the APHA and Defra, to investigate the risk to human health of AI H5N1 in England. Between 1 October 2022 and 15 December 2022, UKHSA health protection systems have recorded 2,085 human exposure episodes of a person being directly exposed to an infected bird, with no detection of AI viruses in humans in 2022 (UKHSA, 2022). There have been no severe human cases associated with H5N1 virus detected in the UK. There is insufficient information to judge the risk of asymptomatic or mild disease due to the limited testing in human contacts with infected birds (UKHSA, 2022).
3.1.2 Symptoms of avian influenza infection in humans
In humans, AI symptoms vary, depending on the virus strain. Symptoms range from a mild flu-like illness, sometimes with conjunctivitis (red, sore, discharging eyes), diarrhoea and abdominal pain, to a severe respiratory illness with breathing difficulties and pneumonia. Human infections may be fatal (NHS, 2018).
The clinical symptoms associated with LPAI infections in humans included: conjunctivitis, mild upper respiratory tract symptoms, lower respiratory tract symptoms or disease, encephalitis, pneumonia, severe pneumonia with respiratory failure, and multi-organ failure. A few deaths have also been associated with LPAI strains (CDC, 2022c).
For HPAI, confirmed human cases of H5 subtypes were associated with mild upper respiratory tract symptoms, lower respiratory tract disease, severe pneumonia with respiratory failure, encephalitis, and multi-organ failure. For human cases from HPAI H7 subtypes, symptoms include: conjunctivitis, mild upper respiratory tract symptoms, lower respiratory tract disease, severe pneumonia with respiratory failure, and multi-organ failure (CDC, 2022c). As noted in Section 3.1, there has been significant case fatalities associated with H5 and H7 AI viruses.
3.1.3 Incubation period in humans
The incubation period in humans is generally 3-5 days but can be longer (NHS, 2018). The UK Health and Safety Executive recommends that anyone who has been in contact with infected birds or their faeces and who develops a flu-like illness should seek medical attention (HSE, 2018).
3.1.4 Infectious dose in humans
Although it is unknown what the infectious dose of AI is for humans, several studies have investigated oral and intranasal inoculation of AI in animals (O’Brien et al., 2021). A study by Bertran and Swayne in 2014 in which ferrets were exposed to different HPAI viruses (H5 and H7 subtypes) through consumption of infected chicken meat, showed that the dose of virus needed to infect ferrets through consumption was much higher (108.9-109.2 EID50 (50% Egg Infectious Dose) compared to 107 EID50) than via respiratory exposure and varied with the virus strain (Bertran and Swayne, 2014).
A 2012 study by Reperant et al showed that intragastric inoculation of domestic cats at a level of 107.8TCID50 (50% Tissue Culture Infective Dose) resulted in fatal systemic infection (Reperant et al., 2012). In 2011, Shinya et al showed that the inoculation of hamsters with H5N1 directly into the digestive tract at a level of 107.1-107.3 TCID50 allowed the virus to enter the bloodstream through the digestive lymphatic system (Shinya et al., 2011).
Since there is a lack of data on AI prevalence and concentration in poultry products and the dose-response relationship in humans, assumptions are made during modelling when performing quantitative risk assessments (Bosch et al., 2018). To support the 2010 FDA/FSIS risk assessment, a dose response relationship was modelled using dose data (Bauer et al., 2010). Their results suggesting that the human EID50 for intranasal exposure could range from approximately 107.8 - 109.5 EID50 (Bauer et al., 2010).
3.2 Avian Influenza incidents in poultry recorded by FSA
To support a One Health approach, detections of AI in livestock are reported to the FSA whether or not they pose a direct food safety risk. Table 2 provides detail on the number of AI incidents recorded by the FSA per year since 2014 through to December 2022. Despite the increase in incidents reported to the FSA by UK animal health agencies, only five incidents related to poultry and their products have been referred to the FSA Microbiological Risk Assessment team for comment or review (2 in late 2019, and three in November 2021).
Table 2: Avian influenza reported to the FSA
Year | AI-related livestock incidents recorded by FSA |
---|---|
2014 | 2 |
2016 | 2 |
2018 | 1 |
2019 | 2 |
2020 | 12 |
2021 | 54 |
2022 (to 5 December) | 172 |
The risk pathway considered in this risk assessment is illustrated in Figure 1. Further detail on what was considered for the three specific products (commercial poultry, game birds, and hen eggs) in scope for this work are provided in the following sections. Since the size of the population of backyard poultry in the UK is not known, nor is it understood how often poultry products from this population is consumed (uncertainty), this pathway was not considered separately. It is highlighted in Section 5 the most related pathways where consideration of backyard poultry might also fall under.
Figure 1: Overall risk pathway from the farm or field through to consumption of poultry products
4.1 Commercial poultry
The risk pathway, through to the processing of the poultry meat, considered for AI-infected flocks of commercial poultry is illustrated in Figure 2.
Figure 2: Risk pathway for avian influenza infection from commercial poultry through to the processing stage.
4.1.1 Overview UK poultry meat production
The number of poultry slaughtered each year in the UK (including broilers, turkeys and boiling fowl) has been increasing from 2010 through to 2021, although the effect of the AI outbreaks led to a decrease in 2022 (Defra, 2023b, 2022b). Poultry slaughtering and poultry meat production in the UK is estimated from the number of chicks placed by hatcheries and day old chick imports combined with advice on the life-span and mortality (Defra, 2022b). Total UK poultry meat production went down by 0.8% at 181.7 thousand tonnes between October 2021 and October 2022 (Defra, 2022b). Although the number of poultry availability in the next month is indicated by the number of eggs set each month, consumer demand strongly affects broiler chick and turkey poultry placing in the months running up to Christmas (Defra, 2022b).
Figure 3: Number of Poultry slaughtered per year in the UK. Boiling fowl includes spent hens and spent breeders. Data from (Defra, 2023b)
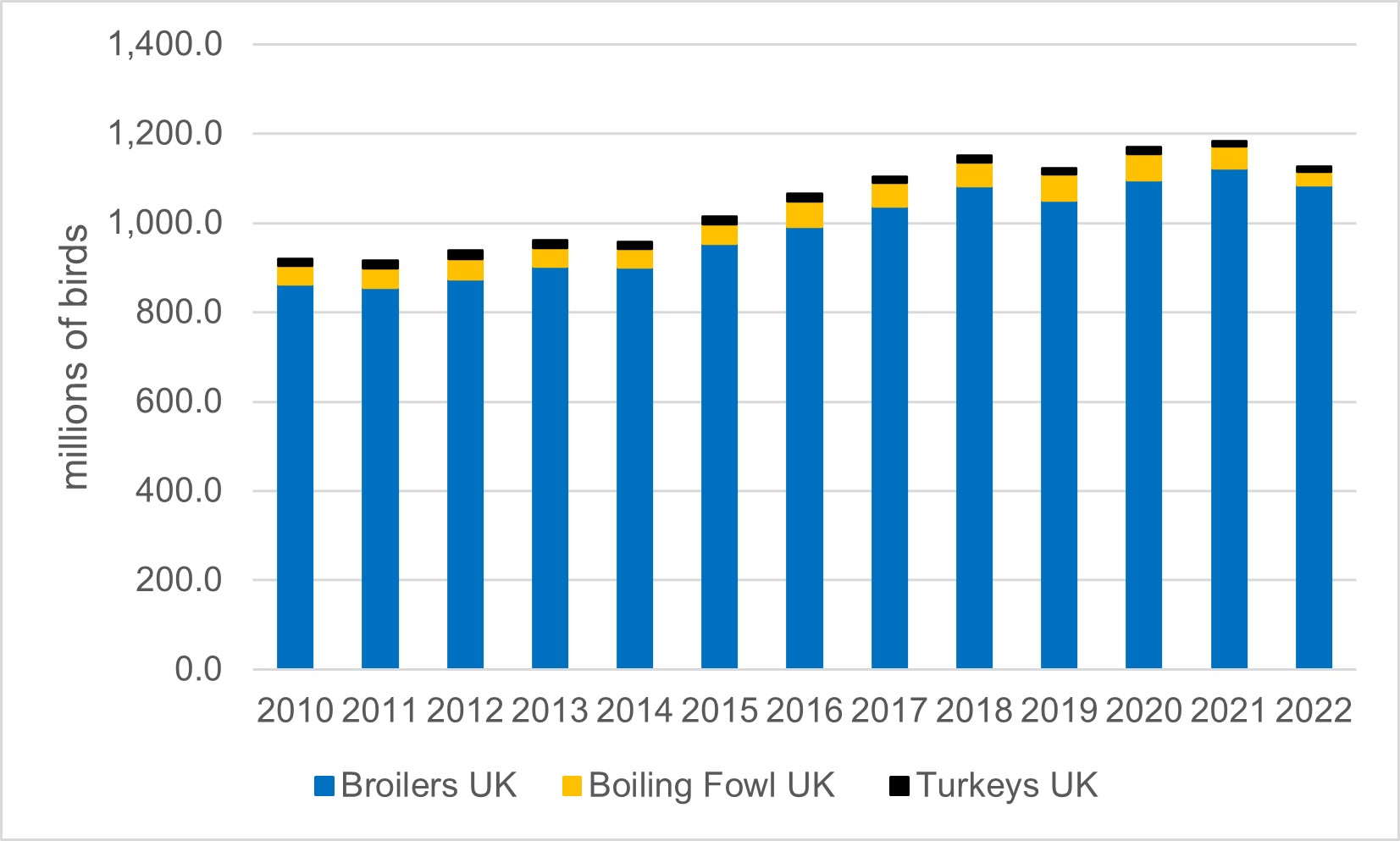
4.1.1.1 Commercial duck and geese production
Although the commercial poultry industry is dominated by the production of chicken and turkeys, there has been a steady increase in the commercial production of other poultry, such as ducks and geese, in recent years. The duck industry, in particular, has expanded to now produce an estimated 16 million birds a year in the UK. These are mainly ducks of the Pekin variety. Approximately 95% are intensively reared and 5% are free range (Barrow et al., 2021). Although primarily produced for their meat, duck eggs are also consumed, and duck feathers are sought after for the filling of home products such as pillows or duvets.
The commercial production of both ducks and geese is included in the legislation described in section 1.5 for the control of AI (The Avian Influenza and Influenza of Avian Origin in Mammals (England) (No.2) Order 2006, 2006). Therefore, commercial producers of these bird types must follow all measures to reduce the risk of transmission and deal with outbreaks, including the culling of positive flocks. However, as non-indicator species, which may not show signs of infection as clearly as chickens, inspection of the carcass may not be sufficient to rule out disease, particularly for LPAI infection (APHA, 2022a) (uncertainty). There is a lack of evidence regarding the clinical signs of HPAI infection in non-indication species, however, some strains have been reported to cause mortality in domestic ducks (Spackman, 2020) (uncertainty). It is assumed that commercially reared ducks will act similarly to wild ducks when infected with AI, as described in Section 4.2 (uncertainty).
4.1.2 Probability of AI in commercial poultry sent for slaughter
Initial clinical presentation of HPAI infection in poultry (chicken and turkeys) might be sudden death, with little or no other preceding clinical signs or gross pathology (More et al., 2017). However, there may be cases of acute disease presenting with signs of systemic infection that could include respiratory distress, coughing, sneezing, cyanosis (blue discolouration) of combs and wattle, haemorrhages on the shank, bleeding from the nares, diarrhoea, circling, incoordination, and death (Abd El-Hack et al., 2022).
Other clinical signs described are oedema (swelling) of the head, dullness, a loss of appetite, or depression (“NADIS Animal Health Skills - Avian Influenza”). Nervous system signs would include ataxia, paralysis of the wings and paddling movements of the legs (More et al., 2017).
An investigation by Post at al. (2012) suggested that for LPAI, no clinical signs were observed. Another study found that LPAI infection in chickens and turkeys manifests with mild respiratory distress and reduced egg production; however tracheitis, sinusitis, air sacculitis, nephritis, ovaritis, and oviduct lesions with egg peritonitis in layers can be observed sometimes (Abd El-Hack et al., 2022).
4.1.2.1 Viral distribution and titre in poultry issue
Post et al. (2012) investigated HPAI and LPAI viral load and manifestation in chickens, demonstrating that H7N1 HPAI infected chickens developed illness with depression that led to death. Their qPCR data showed high amounts of viral RNA load in all organs tested (lung, trachea, ileum, and brain). The most significant difference between HPAI and LPAI RNA load was found in lungs, with LPAI detected from the lung for at least a week. It was summarised that, although both HPAI and LPAI could be detected in a broad range of tissues, the pathogenicity and mortality differences between them could originate from differences in virus replication and the resulting host responses in vital organs (Post et al., 2012). However, another study has reported that the amount of LPAI virus in muscles of poultry (including turkeys and quail) experimentally infected ranged from 100.44 to 101.6-102 /g of tissue, as summarised in the EFSA report (EFSA et al., 2018). Another study has detected LPAI H9N2 virus at 103.5/g from trachea (Shibata et al., 2018). These studies provide evidence that LPAI can be isolated from the muscles and organs of experimentally infected poultry; the presence of LPAI in muscle tissue in naturally infected birds has not been demonstrated in published literature.
Experiments performed by Pantin-Jackwood et al. (2017) demonstrated that turkeys infected with dose of 103 EID50 LPAI, have shown clinical sign of respiratory disease, mild lethargy and infraorbital swelling, with no mortality. Additionally, HPAI infection in turkeys have presented differently to chickens, with more neurological signs and no haemorrhagic lesions present (Pantin-Jackwood et al., 2017).
Tumpey at al. (2002) showed that HPAI H5N1 isolated from duck meat was highly pathogenic and able to replicate in chicken following intravenous and intranasal inoculation (Tumpey et al., 2002).
Infection of the liver in chickens is also a concern due to the consumption of chicken liver pate. Several HPAI and LPAI strains have been detected in the liver of experimentally infected chickens (Post et al., 2012; Yamamoto et al., 2017). The initial viral titre in liver was 104.3 to 106.5 EID50/ml. Liver samples from birds experimentally infected with HPAI H5N1 have been shown to be positive for virus after 20 days when stored at 4°C and 3 days when stored at 20°C (Yamamoto et al., 2017).
4.1.2.2 Probability of virus present in meat of slaughtered birds
HPAI induces a systemic infection in the host – an infection affecting the whole bird in multiple organs. HPAI viral replication causes high titres of infectious virus in internal organs (for example liver, heart) and also in muscle and other tissues, in addition to respiratory and gastrointestinal tracts. (ACMSF, 2015; Harder et al., 2016).
Golden et al. (2009) have used a state-transition model to investigate the probability that an infected flock with HPAI H5N1 will remain undetected until slaughter, considering three possible states, susceptible (not infected), infected, and dead, and the transition probabilities that would predict the movements between those states (Golden et al., 2009). They predicted a high probability (0.94) that a flock infected with HPAI H5N1 would be detected before being sent to slaughter (Golden et al., 2009). The probability of detection was inversely related to the length of the non-detection window (the time between infection and the flock being identified as infected), that is a function of the effective contact rate, latency, bird mortality rate, and daily mortality threshold (Golden et al., 2009).
HPAI can be detected in the muscle and other tissues of poultry, in addition to the respiratory and gastrointestinal tract (ACMSF, 2015). However, it is likely that most flocks infected with HPAI will be detected and culled before entering the UK food chain, since clinically affected poultry will be detected and excluded at the farm or upon ante mortem inspection at the processing plant. Post-mortem clinical signs of HPAI infection at post mortem inspection include: lesions that may include cyanosis and oedema of the head, comb, wattle, and snood (turkey); ischemic necrosis of comb, wattles, or snood; oedema and red discolouration of the shanks and feet due to subcutaneous ecchymosis haemorrhages; petechial haemorrhages on visceral organs and in muscle; and blood tinged oral and nasal discharges (“Avian Influenza - Poultry,” 2022).
Surveillance data revealed that HPAI H5N1 virus was detected in imported frozen duck meat and on the surface and in internal contents of contaminated eggs. Experimentally, HPAI virus was detected in breast and thigh meat, and blood and bones, as well as in eggs of HPAI infected chickens.
LPAI infections in chickens either do not present or very rarely present any clinical signs. Both experimental infection studies (Bergervoet et al., 2019; Roy Chowdhury et al., 2019) and field observations (Bertran et al., 2018) suggest that LPAI viruses are primarily restricted to the respiratory and gastrointestinal tract. As a result of that, it is agreed with the conclusion of the 2018 EFSA report (EFSA et al., 2018) that the virus is very unlikely to be disseminated systemically, although it should be noted that turkeys are more susceptible than chickens and experimentally-infected turkeys may exhibit some systemic infection (EFSA et al., 2018).
Gonzales et al. (2018), on behalf of EFSA, assessed the probability of exposure to LPAI and subsequent infection of the consumer via raw poultry meat and raw table eggs (EFSA et al., 2018). They concluded that the probability of exposure following an infection of LPAI in birds via raw poultry meat containing this virus is negligible for commercial poultry (EFSA et al., 2018). However, it is unlikely that a bird infected with LPAI will be detected via ante-mortem inspection as diagnosis is dependent on the presence of clinical signs (EFSA et al., 2018). Also, the presence of the LPAI virus in muscle tissues is not always associated with the presence of microscopic lesions, and at the high speed of slaughter lines detection of the lesions and faecal contamination of the carcasses by the visual post-mortem inspection can be unidentified (EFSA et al., 2018).
In birds infected with the LPAI virus, it is rarely found in the blood. Therefore, meat would be unlikely to contain high levels of virus and the likelihood of infection to humans in this case is very low (More et al., 2017). Similarly, other products of poultry origin, such as processed meat, are not considered as significant sources for human infection, as they are designated for further treatment (for example, heat processing) (More et al., 2017). Commercial turkeys are highly susceptible to LPAI viruses, often showing increased mortality and are more likely to show clinical signs of respiratory distress, sinusitis, and poor performance (Ngunjiri et al., 2021).
In summary, given the veterinary inspection process in slaughterhouses and clinical signs in the flocks (for example reduced egg production), it is unlikely that HPAI-infected birds will be sent for slaughter. The chances are higher for LPAI-infected flocks since clinical signs may be less acute and signs of infection are less likely to be detected by the post-mortem visual inspection.
4.1.3 Probability that AI present in poultry products survives the retail and consumer behaviour stages
4.1.3.1 Shelf-life of poultry products
According to the guidance on temperature control in food premises, fresh pre-packed poultry should be labelled and kept at -2°C to +4°C (“Guidance on Temperature Control in Food Premises,” 2013). Defra’s “Poultry meat Quality Guide” advises that fresh poultry is to be kept at temperatures not below -2°C and not higher than 4°C (Defra, 2011). Their definition of ‘frozen poultry’ is poultry meat that must be frozen as soon as possible within the constraints of normal slaughtering or cutting procedures and kept at temperatures no higher than -12°C, where this temperature must be stable and maintained (Defra, 2011). ‘Quick-frozen’ poultry meat is defined as a quick-freezing method using authorised cryonic media (air, nitrogen or carbon dioxide) where the zone of maximum crystallisation is crossed as rapidly as possible and the resulting temperature is no higher than -18°C (Defra, 2011).
For storage at home, fresh poultry, such as chicken and turkey, can be stored at chilled temperatures (recommended -1°C to +2°C, maximum of 8°C) for 4-7 days if packed in air or extended to 10 days if modified atmosphere packaging is used (Air Products, 2018). Fresh poultry can be frozen by the consumer for up to 6 months; this length of time is determined by quality concerns of the meat rather than food safety issues (Love Food Hate Waste, 2022).
Although the recommendation is for poultry meat to be kept at the temperatures ranging between -2°C to +4°C, the national requirements (Temperature Control Requirements) of the Food Safety and Hygiene (England) Regulations 2013 in England and the 2006 Food Hygiene Regulations for Wales and Northern Ireland require food that is likely to support the growth of pathogenic microorganisms or the formation of toxins be held at or below 8°C, or at or above 63°C (Reg (EC) 852/2004, 2004; “The Food Safety and Hygiene (England) Regulations 2013”). Since the regulation require the food to be kept at or below 8°C , it is important to keep the fridge temperature at or lower than 5-6°C to ensure the temperature of the food does not exceed the recommended temperature of 8°C (the air temperatures of the fridge are often few degree lower than a temperature of the food itself) (“Guidance on Temperature Control in Food Premises,” 2013).
4.1.3.2 Survival within poultry products
The normal pH range for chicken breast, thighs and drumstick meat is between 5.54 and 6.1, whilst the moisture content is between 70.3% and 72.8% (Beauclercq et al., 2022; Fernandes et al., 2016). Taking into consideration storage conditions, the pH and water content of the poultry (chicken), and the viral persistence in those conditions (described above), it is very likely that AI will persist for at least 100 days in meat.
Although there is no direct evidence that AI has been transmitted to humans via the consumption of contaminated poultry products, there is anecdotal and experimental evidence that the consumption of uncooked poultry blood or meat has transmitted the HPAI H5N1 virus to carnivorous animals, including tigers, leopards, domestic cats, domestic dogs, and a stone marten (Chmielewski and Swayne, 2011).
If food is not thoroughly cooked or properly handled, there could be some contamination of chicken meat with AI virus, and cross contamination during slaughter cannot be excluded. The conclusion of a study carried out by Dai et al., (2022) highlighted the importance of strict inspection and effective disinfection measures in the supply chain of raw poultry worldwide.
4.1.3.3 AI Survival at freezer and refrigeration temperatures
It is well documented from various studies and sources that HPAI tolerates cold and freezing temperatures well. H5N1 virus was shown to be able to survive for 240 days in feather tissue, 160 days in muscle and 20 days in liver at 4°C (Yamamoto et al., 2017). A study carried out in 2012 proved that a high pathogenic strain H7N1 isolated from chicken, turkey and duck meat was still viable after being kept at 4°C for 135, 90, and 75 days, respectively (Beato et al., 2012). Dai et al. (2022) demonstrated that HPAI was able to survive at various temperatures (-20°C, 4°C, and 25°C) for several days (Dai et al., 2022). This study noted that the lower the temperature, the longer the viability of the HPAI. A study carried out in Pakistan showed that the highly pathogenic H5N1 strain remained viable for more than 100 days at 4°C (Shahid et al., 2009). The infectious virus has also been proven to survive in tissues at 20-22°C for up to 6 days post mortem (Busquets et al., 2010).
LPAI is unlikely to be detected in meat, as routine testing is not currently carried out in non-symptomatic flocks. Some research has demonstrated though that if the LPAI virus is present in the poultry meat, it can survive in this environment with little effect on viability, if stored in chilled or frozen conditions. It has been shown that LPAI virus is able to survive freezing and refrigeration, and those low temperatures do not reduce the concentration or viability of viruses (Gonzales et al., 2018). In the experiment by Ejaz et al., 2007, in broiler-chickens infected with LPAI, H9N2, and frozen at -20°C, virus was detected in: bone marrow and legs after 6 weeks post-storage, neck and wings until 4 weeks post-storage, and breast until 2 weeks post-storage (Ejaz et al., 2007). Nevertheless, lungs and kidney tissues in frozen carcasses infected with LPAI strains, might still harbour infectious virus (More et al., 2017).
It has therefore been widely shown that if AI is present in poultry meat, it can survive chilling and freezing conditions with little effect on the viability of the virus. It has also been shown that in general, low temperatures prolong the survival of the virus in poultry tissue. Therefore, the advice still remains to fully cook poultry (ACMSF, 2015).
4.1.3.4 Effect of cooking on avian influenza virus
A study to determine the survival of AI subtype H5N1 under various physical and chemical treatments by Wanaratana et al. (2010) showed that all three H5N1 reference viruses used in the study totally lost their infectivity when exposed to a temperature of 70°C for 60 min, or 75°C for at least 45 min. A study by Swayne in 2006 found that the reduction in virus infectivity titres was dependent on virus concentration and no HPAI virus was isolated after 1 s of treatment at 70°C. A change in coloration in the meat from pink-tan to white was associated with a loss in recovery of infectious virus, which is important to note for less than thoroughly cooked (LLTC) poultry products.
Samanta and Bandyopadhyay found that after 30mins exposure at 56°C AI was no longer viable. (Samanta and Bandyopadhyay, 2017). However a study by Zou et al, has shown that H7N9 virus can remain infectious at temperature up to 65°C exposed for 5min, pH levels ≥3, and UV light for 20min (Zou et al., 2013). Another study has shown that a HPAI H5N1 strain lost its infectivity after 30min at 56°C and after 1 day at 28°C (Shahid et al., 2009).
AI viruses are considered to be heat labile viruses (Swayne et. al., 2004) so cooking poultry and poultry products thoroughly will destroy them. This indicates that properly cooked poultry meat and eggs do not pose a risk of infection. The evidence from the above studies does indicate that the virus will not persist at higher temperatures. There is a degree of uncertainty here as studies have not been conducted on all the known AI viruses although no information has been found which would suggest that any particular subtype is likely to behave differently in terms of heat stability (uncertainty).
4.1.3.5 Consumption of commercial poultry products
Data from the national diet and nutrition survey (NDNS) on the consumption of chicken and turkey is shown in Table 3 and Table 4 (DHSC, 2013; PHE and FSA, 2020, 2018, 2016, 2014). This data only shows meat consumption without recipes included – that is raw products to be cooked and consumed in the home rather than processed foods such as ready meals, take-aways etc. In the NDNS data, chronic consumption is the amount consumed averaged over four days whereas acute consumption is the amount eaten on one day.
Chicken consumption is by far the most popular poultry eaten with 58.67% of the studied population of 4-64 year olds reporting eating chicken, compared with only 9.08% eating turkey. In infants and children under four, 61.09% are consuming chicken and only 5.70% turkey. A slightly different picture can be seen in the over 65s, although once again chicken is the most popularly consumed poultry meat with 46.49% of respondents consuming it and 8.19% for turkey.
Table 3: Chicken consumption (without recipes) from NDNS data
Age range | % reporting | Chronic Mean ^ | Chronic Max^ | Acute Mean^ | Acute Max^ |
---|---|---|---|---|---|
Infants/children <4 (n=3,480) | 61.09% (n=1353) | 9.88 | 78.02 | 20.99 | 163.73 |
4 to 64 years (n=10,228) | 58.67% (n=4329) | 32.43 | 233.03 | 94.26 | 233.03 |
65+ (n=1,538) | 46.49% (n=543) | 32.00 | 240.00 | 98.00 | 400.00 |
^: grams/person/day
Table 4:Turkey Consumption (without recipes)from NDNS data
Age range | % reporting | Chronic Mean ^ | Chronic Max^ | Acute Mean^ | Acute Max^ |
---|---|---|---|---|---|
Infants/children <4 (n=3,480) | 5.70% (n=219) | 8.63 | 36.13 | 30.69 | 110.82 |
4 to 64 years (n=10,228) | 9.08% (n=934) | 23.65 | 113.60 | 79.05 | 367.13 |
65+ (n=1,538) | 8.19% (n=126) | 80.00 | 150.00 | 86.00 | 290.00 |
^: grams/person/day
Figures for the consumption of offal were also requested, however, due to the very low numbers reporting the consumption of offal, the following caveat was implemented, ‘Consumption or exposure estimates made with a small number of consumers may not be accurate. The number of consumers is less than 60, this should be treated with caution and may not be representative for a large number of consumers.’ The consumption of chicken and turkey offal for adults without recipes (not including processed foods etc) is shown in Table 5. There was no data found for children under 19. Only 0.04% of adults (2 out of 5094 respondents) reported consuming chicken or poultry offal and nobody over 65 years old. There was a slight increase in offal consumption with recipes included, such as pre-prepared pate and cooked giblets but this was still very low at 0.84% in adults and 0.52% in the over 65yr olds.
Table 5: Consumption of Offal -Chicken and Turkey (without recipes) from NDNS data
Age range | % reporting | Chronic Mean ^ | Chronic Max^ | Acute Mean^ | Acute Max^ |
---|---|---|---|---|---|
Adults 19 to 64 (n=5,094) | 0.04% (n=2) | 42.00 | 63.00 | 140.00 | 3.10 |
65+ (n=1,538) | 0.00% | 0.00 | 0.00 | 0.00 | 0.00 |
^: grams/person/day
4.1.3.5.1 LTTC Commercial poultry meat
In the FSA’s flagship official statistic survey Food and You 2, 8,672 respondents were asked how often (always, most of the time, about half the time, occasionally, never) they eat either chicken or turkey when it is pink or has pink or red juices (Table 6). In wave 1 (July – October 2020), the data indicated that while a majority (93%) of respondents who eat chicken or turkey report never eating it pink or when it has pink or red juices, 4% report doing this at least occasionally.
Table 6: How often respondents to the FSA's Food and You 2 survey report eating chicken or turkey meat pink.
Response | % of respondents (n=8,672) |
---|---|
Always | 1% |
Most of the time | 1% |
About half the time | 1% |
Occasionally | 4% |
Never | 93% |
4.2 Game Birds
The risk pathway considered for AI infection in game birds, through to the cleaning and processing of the carcass, is illustrated in Figure 4.
Figure 4: Risk pathway for avian influenza infection from game birds through to the processing stage
4.2.1 Overview of the UK game bird industry
Game birds by definition are wild birds that are hunted for human consumption. In the UK common game birds include pheasant, partridge, and grouse as well as waterbirds such as wild geese and wild ducks.
The game bird industry has an estimated worth of over £2 billion a year and continues to grow in popularity. Shoots can no longer rely on wild birds alone, but more often on reared birds. An estimated 83% of shoots use game birds that have been hand reared before being released into the wild.
This is an old practice, with the use of hand reared pheasants and partridges going back over 100 years (“GFA | Game Farming in The UK”). There are approximately 300 game farms in the UK which release an estimated 50 million hand reared pheasants and partridges into the wild for hunting each year (BASC, personal communication). Eggs for hand reared stock are collected in April and hatched in incubators. Although initially reared indoors, chicks are given access to outdoor runs prior to release into the wild to adapt to natural surroundings. In August, at around 8-10 weeks, they are sold to shoots where gamekeepers release them into the countryside (“GFA | Game Farming in The UK”). The shooting season varies depending on the bird but is mainly a winter activity in rural countryside. Not all birds released are shot, some remain in the wild and help to naturally replenish the stock for the following years shooting season.
Wildfowling is similar to game bird shooting; however, it refers specifically to the shooting of geese and ducks and often occurs in marshes or estuaries. Unlike game bird shooting, it does not use reared stocks to supplement the season. Hunted wild geese and ducks mostly migrate from Scandinavia and the Arctic circle in autumn before returning to breeding grounds in Spring (BASC, 2022).
The shooting season for all game birds is tightly regulated and varies depending on species and individual country legislation. Table 7 below summarises the shooting season for each species. The shooting season for most species is in winter which coincides with a higher prevalence of AI in both commercial and wild birds.
Table 7: Summary of shooting season in the UK by species and region (BASC, Quarry Species and Shooting Seasons)
Species | England and Wales | Scotland | Northern Ireland | Isle of Man |
---|---|---|---|---|
Pheasant | October 1 to February 1 | October 1 to February 1 | October 1 to January 31 | October to January 31 |
Grey Partridge | September 1 to February 1 | September 1 to February 1 | September 1 to January 31 | Protected (ban in force) |
Red-legged Partridge | September 1 to February 1 | September 1 to February 1 | September 1 to January 31 | September 13 to January 31 |
Red Grouse | August 12 to December 10 | August 12 to December 10 | August 12 to November 30 | August 25 to October 31* |
Black Grouse | August 20 to December 10 (Somerset, Devon and New Forest: September 1 to December 10) | August 20 to December 10 | - | - |
Ptarmigan | - | August 12 to January 31 | - | - |
Duck and Goose inland | September 1 to January 31 | September 1 to January 31 | September 1 to January 31 |
September 1 to January 31 - Ducks July 1 to March 31 - Geese |
Duck and Goose below HWM (see below) | August 12 to January 31 | August 12 to January 31 | September 1 to January 31 |
September 1 to January 31 - Ducks July 1 to March 31 - Geese |
Common Snipe | August 12 to January 31 | August 12 to January 31 | September 1 to January 31 | September 1 to January 31 |
Jack Snipe | Protected | Protected | September 1 to January 31 | Protected |
Woodcock | October 1 to January 31 | September 1 to January 31 | October 1 to January 31 | October1 to January 31 |
Golden Plover | September 1 to January 31 | September 1 to January 31 | September 1 to January 31 | Protected |
Coot/Moorhen | September 1 to January 31 | September 1 to January 31 | Protected | Protected |
4.2.2 AI in game birds on the farm or field
There is currently no active surveillance or testing of wild game birds for either HPAI or LPAI in the UK (uncertainty). However, some inference can be made from the increasing number of cases of infection detected in reported dead wild birds. Surveillance of wild birds is primarily carried out by patrols by wardens on wild bird reserves, who collect dead birds for testing, and by reports of dead wild birds by members of the public. The numbers of positive findings have increased significantly from 278 in 2021 to 945 in 2022 (APHA, 2022b). The total numbers of positive birds from these cases have increased from 459 in 2021 to 1513 in 2022 (APHA, 2022b). The breakdown of species affected is shown in Figure 5.
Figure 5: The number of avian influenza positive findings in wild birds in 2021 and 2022 broken down by species. Figure generate from data in (APHA, 2022b)
4.2.2.1 Likelihood of detection of clinical signs of HPAI and LPAI in game birds
Clinical signs of HPAI in gallinaceous species of game birds (for example pheasants and partridges) include lethargy, ruffled feathers, bright green faeces and sudden mortality. Several species of birds also show neurological signs such as ataxia (Brookes et al., 2022). LPAI infection in gallinaceous species varies from subclinical to clinical signs of conjunctivitis, and/or mild respiratory and neurological clinical signs. Severe neurological signs are unique to HPAI infection and with LPAI under experimental conditions rarely causing mortality. This makes LPAI infected birds harder to detect (Bertran et al., 2014).
Wild aquatic birds such as ducks and geese are a natural reservoir for AI. Some subtypes, especially LPAI, are highly adapted to these species of birds and therefore infection tends to be subclinical (APHA, 2022a). There are some knowledge gaps with regard to HPAI in these birds. Some subtypes also do not show clinical signs of infection, however, some strains of HPAI have been reported to cause mortality in domestic ducks (Spackman, 2020). Clinical signs have also been observed in wild Anatidae infected with the current circulating strains of AI (APHA, unpublished) (uncertainty).
The severity of clinical signs in gallinaceous species caused by HPAI make infected birds less likely to be shot, either due to the rapid mortality or the unlikelihood that they will fly during the shoot. However, LPAI in these birds and both HPAI and LPAI in aquatic birds is less likely to cause obvious clinical signs (uncertainty).
4.2.2.2 Restrictions on hunting/capture in AI Prevention Zones
Shooting of game birds is not affected by the controls of AI outbreaks within commercial poultry premises. There have however been exceptions during large outbreaks of H5N1 in wild birds, where shooting has been stopped within controlled zones to reduce the movement of infected birds (GOV.UK, 2022b). The housing order for an Avian Influenza Prevention Zone, introduced on November 7 2022 for poultry and captive birds in the whole of England, was amended on January 9, 2023 to restrict the movement of game birds until 21 days after they’ve been “caught up”, referring to the practice of gathering together wild game birds to be held in captivity for the purpose of restocking supplies of game or any breeding programme for the production of such birds (GOV.UK, 2022c).
For HPAI there is usually a Protection Zone (PZ) out to 3km from the IP and a further Surveillance Zone (SZ) out to 10km. Hunting is permitted within these zones, however, movements of birds and bird products are prevented except under licence and gamebird releasing is specifically banned (APHA, 2022a). This has resulted in an estimated 40% reduction of reared game birds being released into the wild for the 2022 shooting season (BASC, unpublished).
4.2.3 AI in game birds during processing stage
4.2.3.1 Likelihood of AI detection before meat released
The likelihood of detection of AI in captive game birds depends on the size of the game establishment and the age of the birds. All holdings keeping 50 or more birds, including game birds that are only housed for part of the year, must register with the government in England, Wales, and Scotland. In Northern Ireland, flocks of all sizes must be registered. Gamebirds, in pens awaiting release into the wild will therefore be required to adhere to regulations in control areas and can be monitored for signs of disease (GFA, 2021). Once old enough to be released, there is no surveillance of game bird populations in the wild so detection at this point is possible only if disease has caused mortality or clinical signs severe enough to see (for example birds too sick to fly).
Those who shoot game birds for human consumption, such as hunters, are recognised as primary producers according to EU food hygiene regulations. Primary wild game products are in-feather game that has not undergone food processing. There are three main routes through which these products enter the food chain for human consumption, summarised in Table 8.
Table 8: Regulations for the sale of game birds in the UK for main routes into food chain for human consumption
Route 1 | Route 2 | Route 3 | |
---|---|---|---|
Use of game bird | Private domestic use | Direct supply of small quantities to the final consumer or local retailer | Supply to retailers through Approved Game Handling Establishment (AGHE) |
Source of game brid | Primary Producer (for example, hunter) | Primary producer (for example, hunter) | Primary Producer (for example, hunter) |
Regulations | Does not require adherence to EU food hygiene regulations | Does not require adherence to EU food hygiene regulations | Approval by FSA or equivalent. Adherence to EU regulations. Carcass subject to specialist and veterinary examination. Records for traceability. |
Firstly, they can be kept for domestic use, where they are only used for domestic consumption by the primary producer or given to friends and family of the primary producer. Domestic use does not require adherence to EU food hygiene regulations as it is not being consumed as part of a food business operation (FSA, 2015). Secondly, the primary producer can directly supply small quantities of in-feather game to final consumers or local retailers, which also does not require adherence to EU food hygiene regulations (FSA, 2015).
Finally, the producer can supply in-feather game indirectly to consumers or local retailers, but this must be through an Approved Game Handling Establishment (AGHE) (FSA, 2015). To sell to an AGHE, primary producers must register with their local authority, comply with the food business operator’s responsibilities under both the general hygiene requirements for primary production, Regulation 852/2004 (Reg (EC) 852/2004, 2004), and the specific provisions in Regulation 853/2004 for the initial handling of wild game intended for subsequent supply to an AGHE (Reg (EC) 853/2004, 2004). This includes the requirement that a trained person is present to inspect the game when it is shot, and that temperature controls and hygienic transport is used for storage and transport.
To act as an AGHE, establishments must be approved by FSA, Food Standards Scotland (FSS), or by the Department of Agriculture and Rural Development (DARD) depending on geographical location. They must adhere to various other procedures and retained EU regulations such as 852/2004 and 853/2004 and waste disposal Regulation (EC) No.1069/2009. EU Regulation 852/2004 includes the requirement of storage of in-feather game in a regulated game larder which is required to have appropriate ventilation and temperature control (Reg (EC) 852/2004, 2004). It must be regularly cleaned and protected against animal and pest contamination. EU regulation 852/2004, stipulates that wild game must be cooled to no more 4⁰C within a reasonable time after killing on a continual cooling curve and maintained at these temperatures or below. Chillers must be cleaned regularly and not overfilled. Furthermore, they are subject to official veterinary controls and inspections and can only process game that has been initially examined and approved by a trained specialist after a shoot. Finally, they are required to keep detailed records for traceability to identity where the game has come from (geographical location) and verify the specialist has been properly trained to inspect the products (FSA, 2015).
Due to the regulations to be an official AGHE or sell to an AGHE, this route of game birds into the food chain for human consumption has the lowest possibility for AI transmission. Examination of carcasses by trained specialists should reduce the likelihood of HPAI infected birds being sold, due to the clear pathology caused by this type of infection - such as inflammation of the sinuses, trachea and air sacs. Oedema of the head and discolouration of the skin may also be seen, and birds will likely show signs of dehydration and their muscles may be congested. Haemorrhages on the lining of the proventriculus, the glandular stomach, can also occur (NADIS, 2016). Similarly, the refrigeration and hygiene controls should reduce cross contamination and the requirement for traceability means carcasses can be traced back to infected areas.
Game birds, however, used for private domestic use or sold directly in small quantities to local retailers will not have undergone these checks and there is no regulation on the processing stages, such as defeathering in the home, so potentially have higher likelihood of transmission (uncertainty).
The exact number of game birds shot each year and consumed through unregulated routes is unknown (uncertainty). However, estimates provided by The British Association for Shooting and Conservation (BASC), suggest 37.5% of the 50 million game birds released each year are shot, 97% of which are consumed. 35% of those consumed will go to restaurants and shops through an AGHE and 62% are consumed domestically or are sold through non-AGHE’s (therefore non-regulated) (BASC, personal communication). Due to the restrictions on release of game birds in PZ’s BASC also estimate that 40% less fewer birds were released into the wild for shoots throughout 2022. Based on these estimates the numbers of game birds consumed in the UK through various routes are calculated in Table 9, for a typical year and 2022. In 2022, an estimated 3.94 million game birds were sold though as AGHE while 6.77 million game birds were sold through non-AGHEs or used for home consumption.
Table 9: Estimate of number of game birds consumed in the UK on a typical year versus 2022 through various routes of sale
Year | Released for shooting (million) | Number shot (million) | Sold to Restaurants/shop through AGHE (million) | Non-AGHE sale and home consumption (million) | Not consumed (million) |
---|---|---|---|---|---|
Typical year | 50 | 18.75 | 6.56 | 11.28 | 0.56 |
2022 (due to AI restrictions) | 30 | 11.25 | 3.94 | 6.77 | 0.34 |
To conclude, the likelihood of AI infected game birds being detected before reaching the home for processing and consumption is greater if acquired thought the AGHE route. The regulations, including carcase inspection, storage, transport, and processing of carcases as well as product traceability all further reduce the likelihood of cross contamination and infected material reaching the consumer home. However, due to the lack of data collected on game birds acquired through hunting the number of birds consumed through the non-AGHE route can only be estimated (uncertainty).
4.2.3.2 Viral distribution and titre in game birds
As AI is a respiratory pathogen the transmission routes of AI from birds to humans is believed to be through direct, close contact with respiratory secretions. However, the virus has been found in the feathers, blood, organs, bones and skin of infected game birds making these other possible routes of transmission.
4.2.3.2.1 Feathers
In ducks, the titre of HPAI H5N1 in the feather follicle has been shown to be high enough to be infective to other birds and was shown to persist for days to weeks, at various temperatures, after the bird has been dispatched and the feather plucked, increasing the risk feathers may function as fomites (Yamamoto et al., 2010). A possible reservoir of the virus in the feather of water birds such as ducks or geese is preen oil, a secretion from the preen gland in these birds which they spread across their feathers during preening. Preen oil has been shown to accumulate various pathogens, including high levels of H5N1 (Karunakaran et al., 2019).
The levels of H5N8 in feathers and cloacal swabs were compared in positive flocks of ducks and geese. The viral loads were found to be 103 higher in the feather samples compared to the cloacal swabs. This result was consistent across all flocks (Gaide et al., 2021).
No publications could be found through extensive literature search that looked for levels of LPAI in game bird feathers (uncertainty).
What these publications demonstrate is that a range of AI strains persist in the feathers of various game birds at high levels, highlighting a potential risk of transmission to humans (uncertainty). The defeathering process requires close contact with the bird and the process of defeathering can also lead to airborne infectious particles of AI (Bertran et al., 2011).
4.2.3.2.2 Organs and blood
HPAI has been found in a range of organs in game birds including the cloacal bursa, spleen, liver, kidney, heart, brain, lung, pancreas and skeletal muscle (Bertran et al., 2014). The presence in the organs is believed to be due to the ability of HPAI to infect endothelial cells resulting in viremia and subsequent systemic spread (Vreman et al., 2022). In contrast, as discussed in 4.1.2.1, LPAI infections typically are restricted to the respiratory and intestinal systems and are rarely seen in other organs (Vreman et al., 2022).
4.2.3.2.3 Meat
It is well established that HPAI, but not LPAI, can be found in the meat of chickens. Experimental infections have been have carried out to directly compare HPAI and LPAI dissemination in chicken meat. Whereas HPAI caused systemic spread to the breast and thigh meat, LPAI infection was found to be localised to the respiratory and GI tract (Swayne and Beck, 2005).
Although similar studies could not be identified in the literature for game birds, there have been reports of HPAI found in the meat of various game birds such as ducks (Wu et al., 2020).
In summary, the presence of HPAI in the feathers and organs of game birds is of particular concern in carcasses used for domestic purposes. Without regulations necessitating a properly functioning game larder, with good ventilation and hygiene practices, there may be a greater risk of transmission from the processing of these birds (uncertainty).
4.2.4 AI in game birds during retail and consumer stages
4.2.4.1 Survival in meat from game birds
There is limited research into the survival of avian influenza in meat from game birds, although a 2012 study by Beato et al. determined that HPAI H7N1 could survive for up to 75 days in duck meat kept at 4°C (Beato et al., 2012). It is assumed that AI survival within meat from game birds will behave similarly to its behaviour in poultry meat (Section 4.1.3.2).
4.2.4.2 Effect of cooking game meat on AI
There is limited research into the effect of cooking on the inactivation of game bird meat, although it is considered likely that the this will be similar to the effect described for poultry meat discussed in Section 4.1.3.4.
4.2.4.3 Consumption of game birds
Data from the national diet and nutrition survey (NDNS) on the consumption of duck and other game birds are provided in Table 10 and Table 11(DHSC, 2013; PHE and FSA, 2020, 2018, 2016, 2014). The majority of duck consumption relates to commercially reared poultry as described in section 4.1.1.1. We are using this data as there is limited equivalent data available on wild game duck. This data only shows meat consumption without recipes included – that is raw products to be cooked and consumed in the home rather than processed foods such as ready meals, take-aways etc. In the population ranging in age from 4-64 years old, 1.28% report eating duck. Other game is very rarely reported being consumed by only 0.17% of the participants. In infants, duck is rarely consumed with only 0.26% reporting consuming it and a negligible 0.08% reported consumption of other game. A slightly different picture can be seen in the over 65s, as this is the most popular age group for consuming duck and game, with 1.82% of participants consuming duck and 0.46% consuming other game. With the figures for duck and game being so low, we should note that consumption or exposure estimates made with a small number of consumers may not be accurate. When the number of consumers is less than 60, this should be treated with caution and may not be representative for a large number of consumers.
Table 10: Duck consumption (without recipes) from NDNS data
Age range | % reporting | Chronic Mean^ | Chronic Max^ | Acute Mean^ | Acute Max^ |
---|---|---|---|---|---|
Infants/children less than 4 (n=3,480) | 0.26% (n=10) | 11.10 | 34.00 | 34.00 | 74.50 |
4 to 64 years old (n=10,228) | 1.28% (n=132) | 24.34 | 119.77 | 89.42 | 286.74 |
65+ (n=1,538) | 1.82% (n=28) | 20.00 | 58.00 | 79.00 | 230.00 |
^: grams/person/day
Table 11: Other game birds (not duck) (without recipes) consumption from NDNS data
Age range | % reporting | Chronic Mean^ | Chronic Max^ | Acute Mean^ | Acute Max^ |
---|---|---|---|---|---|
Infants/children less than 4 (n=3,480) | 0.08% (n=3) | 9.20 | 10.20 | 33.33 | 33.33 |
4 to 64 years old (n=10,228) | 0.17% (n=18) | 27.61 | 44.06 | 103.61 | 172.22 |
65+ (n=1,538) | 0.46% (n=7) | 47.00 | 79.00 | 150.00 | 260.00 |
^: grams/person/day, *: n is total number of respondents.
4.2.4.3.1 LTTC game bird meat
In the FSA’s flagship official statistic survey Food and You 2, 4,227 respondents were asked how often (always, most of the time, about half the time, occasionally, never) they eat duck meat when it is pink or has pink or red juices (Table 12). In wave 1 (July – October 2020), the survey found that around two thirds (67%) of respondents who eat duck report never eating it pink or when it has pink or red juices, yet almost a third (31%) eat pink duck at least occasionally; 3% report always eating duck pink, 8% do this most of the time, 4% about half the time, and 16% occasionally.
Table 12: How often respondents to the FSA's Food and You 2 survey reported eating duck poultry meat pink
Response | % of respondents (n=4,227) |
---|---|
Always | 3% |
Most of the time | 8% |
About half the time | 4% |
Occasionally | 16% |
Never | 67% |
4.3 UK Egg Production
The risk pathway, through to packing and processing step, considered for eggs infected with AI reaching the consumer is illustrated in Figure 6.
Figure 6: Risk pathway for avian influenza infection from eggs through to the processing stage.
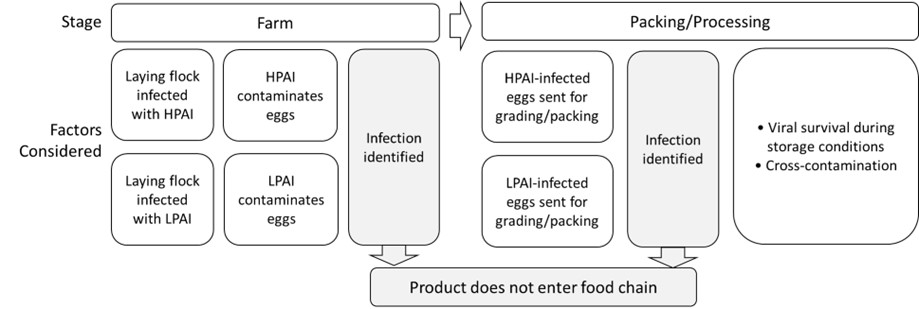
4.3.1 Overview of Egg production in the UK
Based on 2021 industry estimates, the UK consumes 13.5 billion eggs a year. A majority of these eggs are produced within the UK, based on industry estimates of UK egg production at 11.3 billion eggs, with 1.4 billion eggs imported and 405 million eggs exported (British Lion Eggs, 2022). A majority of these eggs, 65%, enter the market at retail as shell eggs while the remainder enter as egg products (17%) or the food service industry and would thus need to adhere to food safety regulations (British Lion Eggs, 2022).
Hen laying flocks may be kept in cages, barns or be free-range; however, housing orders introduced during AI outbreaks to help reduce transmission would affect the amount of free-range eggs that are on the market; the last housing order for all poultry and captive birds in England was introduced on November 7th, 2022. (GOV.UK, 2022c). Typically, eggs are transported from farms to packing centres, where the eggs are graded and packed. Nearly 90% of UK eggs are produced following assurances required by the Lion Code scheme (British Lion Eggs); this assurance scheme introduces extra measures producers must adhere to in order to reduce the levels of Salmonella reaching consumers through table eggs. Eggs that don’t meet Grade A requirements (which may be due to cracks in the shell or dirt on the shell) are classed Grade B and heat treated to remove any potential pathogens before further use (British Lion Eggs); these eggs are therefore out of scope for this risk assessment. Transport times and storage times and the temperatures associated with them are variable. These values were estimated by an expert elicitation exercise which suggested eggs are likely to encounter a temperature of about 15°C during the processing and supply chain and may take over 30 days to reach retail from the farm (EFSA, 2014).
An additional route to market would be small producers selling eggs directly to consumers, either through on-farm sales or at local market stalls. The number of laying hens from small flocks compared to those large enough to be registered with APHA is estimated to be a very small percentage of the egg market. Additionally, these eggs would only reach a local market. The handling of these eggs in terms of storage times and temperatures is not known (uncertainty).
The FSA recommends that eggs be stored in a cool, dry place and ideally in the fridge (FSA, 2022a). Grade A eggs are required to carry a best before date (a maximum of 28 days after laying). Smaller producers that do not need to be registered with APHA must still provide best before dates for their eggs and recommend consumers keep them chilled (“The Eggs and Chicks (England) Regulations 2009”). As best before dates are indicators of quality and not safety, the FSA suggests eggs past their best before date are safe to eat provided they are thoroughly cooked (FSA, 2022a). It is not known how long consumers may keep eggs before consuming (uncertainty).
4.3.2 AI Infections in laying hens on the farm
4.3.2.1 Effect of HPAI infection on laying hen flocks and their eggs
Infection with HPAI in commercial poultry will lead rapidly to presentation of clinical signs, typically within 2-3 days post-infection (see Section 324.1.2). There is often a drop in egg production, which ranged from 10-55% when studied in flocks naturally infected (Lu et al., 2004); in another study, this reduction reached a drop of about 30% in egg production (down from 79% egg production, based on a three day average of percentage of eggs/hen/day) within 3 days of infection in chickens infected experimentally (Swayne et al., 2012). Additionally, HPAI infection may lead to changes in the egg, such as malformed eggs (Qi et al., 2016; Swayne et al., 2012). During an outbreak, researchers found that up to 10% of eggs were malformed, including being “thin-shelled, soft-shelled or abnormally small” (Cappucci et al., 1985).
During HPAI infections, the reproductive tissue of laying hens can be infected (Harder et al., 2016). If the ovary is infected, this can lead to infectious virus being present in the yolk. If the oviduct is infected, virus will also be present in the albumin (egg whites). The virus can be present on the shell of the egg, although it is likely this is due to faecal contamination from passage through the cloaca (EFSA, 2006).
As chickens will likely stop laying eggs around 4 days post-infection due to either clinical progression of disease or death, there is the possibility that eggs laid in the period between infection and clinical detection could contain virus. Experimental infections determined that eggs laid early in the infection did not exhibit virus in or on the egg, but it was found in the last eggs laid (Bauer et al., 2010; Cappucci et al., 1985). Studies from outbreaks of naturally infected flocks estimated 7-57% of eggs may be HPAI positive (Bauer et al., 2010; Cappucci et al., 1985). Another study found that 53% of eggs from hens experimentally infected with HPAI H5N2 contained the virus (Swayne et al., 2012). Available data on viral titres isolated from HPAI-infected eggs and the time of collection post-infection are summarised in Table 13.
Table 13: Avian Influenza viral titres isolated from egg internal contents. Table adapted and updated from Bauer et al., 2010
Reference | Bird species and HPAI strain | Egg product and titre (EID50/ml) | Time taken dpi^ | Type of infection |
---|---|---|---|---|
(Bean et al., 1985) | Hens H5N2 |
White: 105.6 Yolk: 103.6 |
Last eggs laid before death | experimental |
(Promkuntod et al., 2006) | Quails H5N1 | Egg internal contents: 104.6 - 106.2 | Not reported | natural |
(Swayne et al., 2012) | Hens H5N2 |
Shell: 102.99 Albumin: 103.01 Yolk: 102.17 |
1-4 dpi | experimental |
(Uchida et al., 2016) | Hens H5N8 |
Shell: 102.7-5.5 Albumin: 102.2-5.3 Yolk: 101.5-4.4 |
3-4 dpi | experimental |
^dpi: days post-infection
Given the clinical signs of HPAI in infected flocks, it is likely that the infection would be identified, and eggs stopped from reaching the market. However, there is a small window between initial infection and the presentation of clinical signs where contaminated eggs may be released from the farm containing viral loads between 101.5 – 106.2 EID50/ml.
4.3.2.2 Effect on LPAI infection on laying hen flocks and their eggs
Flocks infected with LPAI viruses do not present with as many clinical signs as those infected with HPAI viruses. The only evidence may be ruffled feathers and a slight drop in egg production; it is likely it may not be detected at all (Center for Food Security and Public Health, 2022). LPAI viruses have not been found replicating in as many tissues in birds and are typically restricted to the respiratory and gastrointestinal tract (Harder et al., 2016). This means eggs are less likely to contain active LPAI virus as the reproductive tract is not infected. While some studies have not found LPAI in or on eggs from LPAI-infected flocks (Lu et al., 2004), other evidence suggests that LPAI can be found in the internal contents of eggs, although this has been reported less frequently than finding HPAI in egg internal contents (Cappucci et al., 1985; Pillai et al., 2010). Evidence also suggests that LPAI is found less frequently in eggs from LPAI-infected flocks, with only 1.67% (2/120) of eggs from commercial layers positive for H5N2 LPAI compared to 24.6% (37/156) of eggs positive for the high pathogenicity version of the strain during a natural outbreak (Cappucci et al., 1985). There is still the possibility for faecal cross contamination of the eggshell surface from passage through the cloaca. How the different LPAI viruses may behave in different bird species as far as their ability to contaminate eggs internal contents and the shell is unknown (uncertainty).
4.3.3 AI contamination in eggs during the grading and packing process
As noted above, up to 10% of eggs produced by hens infected with HPAI were malformed, including being “thin-shelled, soft-shelled or abnormally small” (Cappucci et al., 1985). These eggs would be removed from the food chain during the grading process (APHA, 2018). Class A eggs are not allowed to have foreign matter on them, which means eggs with visible faecal contamination, which may carry the AI virus (see discussion in Section 4.4.1), will also be removed from the food chain (APHA, 2018).
4.3.4 AI in eggs during the retail and consumer stages
4.3.4.1 Survival in eggs
Experimental infection with HPAI strain H5N2 in and on eggs provided some estimation of the viral survival during storage times of different lengths and temperatures. When virus was applied on the eggshell and allowed to dry, it could not be re-isolated from the surface after 3-4 hours, regardless of the temperature exposure. The same held true even if the virus was mixed with “droppings” (assumed to be bird faeces). However, active virus could be isolated from the internal contents of the eggs. Virus survived better in the yolk compared to the albumin, and viral titres in both dropped off as either length of storage or temperature increased. At 4°C, virus was still detected after 17 days in both the yolk and albumin, whereas it was only found in the yolk after 17 days at both 15°C and 20°C (de Wit et al., 2004).
4.3.4.2 Evidence for heat inactivation in eggs
Inactivation of AI in eggs at pasteurisation temperatures has been studied using experimentally inoculated egg samples. Swayne and Beck (2004) found that for both HPAI and LPAI H5N2, viral titre was reduced by 90% within 20 seconds at 61°C in both homogenised whole egg and in egg whites. Another study found there was a 5-log reduction in H5N2 HPAI in 34 seconds for homogenised whole egg and in 4 seconds for plain egg yolk at 60°C (Chmielewski et al., 2013). Viral reduction could also be achieved at lower temperatures for longer treatments, ranging from 4.3 minutes for reduction of HPAI in liquid egg at 55°C to 11 minutes for HPAI in whole homogenised egg at the same temperature (Swayne and Beck, 2004).
4.3.4.3 Consumption of eggs
Data from the national diet and nutrition survey (NDNS) on the consumption of eggs by different age ranges are presented in Table 14 - Table 16 (DHSC, 2013; PHE and FSA, 2020, 2018, 2016, 2014). These data include foods consumed “with recipes”, meaning that data is included both when the egg is eaten as an individual item and when it forms part of a recipe. Since AI can be inactivated by thorough cooking of eggs, data on eggs that would be LTTC was also included. In the tables below, “all egg consumption” would include raw, poached, and thoroughly cooked eggs.
Table 14: Egg consumption data for infants and children (less than 5) n=4,227)
Egg consumption | % reporting | Chronic Mean^ | Chronic Max^ | Acute Mean^ | Acute Max^ |
---|---|---|---|---|---|
Raw eggs | 1.4% (n=60) | 2.4 | 34.4 | 4.2 | 30.1 |
Poached eggs | 1.2% (n=49) | 16 | 43 | 58.6 | 112.1 |
All egg consumption | 72.5% (n=3,065) | 9.9 | 100.3 | 29.5 | 232.6 |
^: grams/person/day
Table 15: Egg consumption data for people aged 5 to 64 years. n=14,995
Egg consumption | % reporting | Chronic Mean^ | Chronic Max^ | Acute Mean^ | Acute Max^ |
---|---|---|---|---|---|
Raw eggs | 3.6% (n=541) | 1.2 | 28 | 4.3 | 80.7 |
Poached eggs | 5.4% (n=815) | 30.4 | 210 | 99 | 400 |
All egg consumption | 94.6% (n=14,189) | 22.7 | 870 | 59.5 | 870 |
^: grams/person/day
Table 16: Egg consumption data for UK people aged 65+. n=1,538
Egg consumption | % reporting | Chronic Mean^ | Chronic Max^ | Acute Mean^ | Acute Max^ |
---|---|---|---|---|---|
Raw eggs | 4.8% (n=74 | 1.2 | 10 | 3.2 | 24 |
Poached eggs | 9.1% (n=140) | 24 | 130 | 78 | 150 |
All egg consumption | 94.5% (n=1454) | 23 | 150 | 60 | 280 |
^: grams/person/day
The data reveal that a vast majority of the UK population, in all age groups, consumes eggs. The amount that is eaten raw or poached is much smaller, with the elderly reporting the highest percentage of consumers eating raw and poached eggs. While those aged 5 to 64 years report eating raw and poached eggs less frequently than those that are 65+, they report eating larger servings of these products.
Data from the FSA’s Food and You survey was also reviewed to support evidence on egg consumption in the UK (Table 17; (FSA, 2021)). Self-reported rates of consumption of eggs eaten raw, LTTC and thoroughly cooked indicated that, of the respondents that consume eggs at home, only a small percentage eat raw eggs once a week or more. A higher percentage eat LTTC eggs and thoroughly cooked eggs. 61% of respondents never eat raw eggs, 22% never eat LTTC eggs and 8% never eat thoroughly cooked eggs. Both data sets indicate that when eggs are consumed in the UK, they are most frequently eaten thoroughly cooked.
Table 17: Frequency of eggs eaten raw, LTTC or thoroughly cooked in the UK.
Data from Food and You 2 (Wave 2) survey on the eating habits of UK consumers (FSA, 2021).
Occurrence | Raw (a) | LTTC (b) | Cooked thoroughly |
---|---|---|---|
Every day | 1% | 1% | 2% |
Most days | 2% | 4% | 5% |
2 to 3 times a week | 4% | 12% | 15% |
About once a week | 7% | 24% | 27% |
2 to 3 times a month | 4% | 15% | 16% |
About once a month | 4% | 11% | 15% |
Less than once a month | 14% | 9% | 1% |
Never | 61% | 22% | 8% |
a: eggs that are uncooked for example, in homemade mayonnaise or homemade desserts like mousse or soft meringues
b: eggs that have a runny yolk for example, soft boiled
c: eggs that have a firm yolk for example, hard boiled
4.4 Cross-contamination of AI from birds and poultry products
4.4.1 Survival in faeces
Both HPAI and LPAI is shed in the faeces of both symptomatic and asymptomatic carriers of the disease. It is quite often associated with the further spread and infectivity of the disease – particularly with the wild bird population contaminating water and feed sources.
Experimentally it has been shown that AI might remain infectious in duck faeces within a wide range of days to several weeks depending on temperature conditions. A conservatively estimated expected viral titre in 1g of duck faeces was replicated by spiking duck faeces with tissue culture infective dose (TCID) of 106 TCID50/ml in 1g. The study found that influenza viruses may remain infectious in duck faeces for periods of time ranging from a few days (at 30°C and 20°C) or a few weeks (at 10°C) to several months (at 0°C). The study took into account their previous work where a replicated egg infective dose per g of fresh duck faeces became undetectable after the faeces were dried overnight at room temperature (20°C), while in wet faeces, the virus remained viable for 4 to 6 days at 37°C leading to uncertainty about the virus’ persistence in dried faecal matter (Nazir et al., 2011).
A study held in India showed that the survival time of the virus in faeces was up to 30 days at 4°C and for 7 days at 20°C, however it only persisted for up to 24h and 18h at 37°C and 42°C respectively, in both wet and dry faeces (Kurmi et al., 2013).
When spiking faeces with HPAI H5N8 and LPAI H6N2, differences in persistence were seen between the strains. HPAI persisted until the end of the experimental period, 96 hours, while the LPAI strain was only detected until 24 hours after the material was spiked. In both cases, temperatures ranged from 19 to 22.5 °C in the room where the experiment was performed (Hauck et al., 2017).
As noted in Section 4.3.3, eggs with visible contamination on the shell will be removed during the grading process, reducing the amount of faecal contamination consumers may encounter when handling table eggs. Insufficient cleaning of in-feather poultry or game birds to remove any faecal contamination could be a route of transmission during slaughter and processing of the carcass, particularly in a domestic setting (uncertainty). There is strong evidence that the virus will persist in faecal matter and without thorough cooking and hygienic handling of contaminated products, cross-contamination could occur.
4.4.2 Survival in the environment
Environmental persistence of AI virus has been determined as an important factor for the epidemiology of the virus within wild bird populations and within aquatic habitats. The wild bird population is proven to be an important vector in the spread of the virus to the domestic and commercial poultry industries. Surface water is considered to be a major site of environmental contamination (Keeler et al., 2014).
The majority of studies that have investigated the persistence of AI in the environment have tended to concentrate on the persistence of the virus in faeces. Wood et al., (2010) carried out a study to investigate how the virus persisted in the environment on different surfaces, in different humidity and temperature conditions. They tested the persistence on porous surfaces (soil and faeces) and non-porous surfaces (glass and galvanised metal) (Wood et al., 2010). Using test results and prediction modelling they estimated that HPAI could survive up to 57 days on galvanised metal and 72 days on glass surfaces. HPAI persisted longer without exposure to both UV-A and UV-B simulated sunlight than with exposure. The detrimental effect of UV was markedly higher on the HPAI on the non-porous surfaces. Low temperatures lead to a longer persistence in all cases. Overall, the study concluded that the HPAI H5N1 virus used in the study tends to survive longer in the environment (outside a host) in areas that are relatively colder and have less sunlight.
Brown et al., (2009) investigated AI virus persistence in water at different pH, and temperatures. They found that all the AI viruses tested in the study were, in general, most stable at pH 7.4-8.2 at lower temperatures of <17°C, and in fresh to brackish salinities (salinity ranging from 0 to 20,000 ppm) (Brown et al., 2009). The study by Keeler et al., (2014) showed similar results in that persistence of the virus was longest in waters at temperatures as low as <17°C, neutral to basic pH of 7.0-8.5, and a low salinity of less than 0.5 ppt (Keeler et al., 2014)
4.4.3 Effect of disinfectants on avian influenza
AI can be inactivated by a range of disinfectants at the recommended concentrations (Shahid et al., 2009):
- Formalin (0.2, 0.4 and 0.6% after 15 minutes)
- Iodine crystals (0.4 and 0.6% after 15 minutes)
- Phenol crystals (0.4 and 0.6% after 15 minutes
- Virkon®-S (0.2% after 45 minutes, 0.5 and 1.0% after 15 minutes)
- Surf Excel (soap), Life Buoy (detergent) and Caustic soda (alkali) inactivated the virus at 0.1, 0.2 and 0.3% concentration, respectively, after 5 minutes contact time.
Guan et al., (2015) investigated an effectivity of disinfectant against avian influenza AI during winter months. They have shown that commercial disinfectants, such as Virkon and Accel, when supplemented with antifreeze agent (propylene glycol-PG, methanol-MeOH, or calcium chloride-CaCl2) inactivated AI within 5min at -20°C or 21°C by 106. However, the PG and MeOH did not kill AI alone at those temperatures, but 20% CaCl2 inactivated 105 AI within 10 min. Similarly, Kabir at al. (2021) tested the microbicidal activity of a mixture of quaternary ammonium compounds (QACs) and food grade additive grade calcium hydroxide (FdCa(OH)2) at -20°C using anti-freeze agent (AFA) containing methanol against a variety of viruses, including AI (Kabir et al., 2021). They found that avian influenza was inactivated within 30min of the treatment at -20°C, and within 10min at 1°C (Kabir et al., 2021). Another study investigated survival of HPAI H5N1 against chlorination, and found that free chlorine concentrations (0.52–1.08 mg/L) typically used in drinking water are sufficient to inactivate the virus by >3 orders of magnitude, after 1 min exposure (Rice et al., 2007). An acidic agent, potassium monopersulfate (PMPS) was investigated against AI, and showed that it is able to inactivate the virus at 5000, 2,500, and 1,250 ppm within 30sec, 5min and 15min, respectively (Sonthipet et al., 2018). Ota et al. (2016) investigated the antiviral properties of calcinated egg shell (Egg-CaO) in the form of powder and aqueous solutions against pathogens, including AI (Ota et al., 2016). They showed that AI was inactivated after 1h exposure, and that the powder was effective against AI after both exposure to sunlight for 2 weeks or re-suspension of the powder up to 7 times with water - simulating the harsher conditions in the field rather than the laboratory. (Ota et al., 2016).
4.5 Infectivity of avian influenza in the human GI tract
Influenza virus hemagglutinin (HA) proteins binding to host cell sialic acid-based receptors is known to be a first step in viral invasion of the host, as well as considered to be the first barrier to cross-species transmission (Zhao and Pu, 2022). Avian and human influenza viruses have a different sialic acid (SA)-binding preferences, but only a few amino acid changes in the HA protein are needed to cause a switch from avian to human receptor specificity (de Graaf and Fouchier, 2014). AI viruses have a preference for SA linked to the galactose in an α-2,3 format, whereas human influenza viruses (H1, H2, and H3) prefer SA that are linked in an α-2,6 format (de Graaf and Fouchier, 2014). The human upper respiratory tract lacks SA α-2,3-Gal, which may help explain the species barrier seen between humans and birds in becoming infected with AI.
One component of saliva that can have anti-influenza virus activity is sialic acid-containing molecules. Limsuwat et al. (2014) investigated the role of human saliva against influenza viruses by hemagglutination inhibition (HI) and neutralization (NT) assays (Limsuwat et al., 2014). They showed that human saliva had higher antiviral activity against human influenza viruses compared to an H5N1 avian influenza strain (Limsuwat et al., 2014). They then went on the characterise the sialic acids present in human saliva, identifying that SA α-2,6-Gal was more abundant than SA α-2,3-Gal (Limsuwat et al., 2016). This supports the evidence that human saliva will have lower antiviral activity against AI viruses due to the presence of a greater amount of SA with preferential binding to human-adapted influenza viruses.
Since human cases of AI also report gastrointestinal symptoms, one study has investigated whether AI H5N1 virus can infect and replicate in the human GI tract (Shu et al., 2010). While SA α-2,6-Gal was abundantly expressed along the entire GI tract, they did identify expression of SA α-2,3-Gal, which increased traveling from the ileum to the rectum. Using human ex vivo colon samples, the research group was able to demonstrate infection of the tissue by the AI virus. Increases in viral titre in the supernatant of the ex vivo cells suggested the virus was also capable of replication (Shu et al., 2010). For evidence of active AI infection in the human GI tract, colonic samples of an H5N1 deceased patient were stained for presence of the nucleoprotein viral antigen, which was detected in the tissue. Furthermore, in two other H5N1 patients experiencing diarrhoea as a symptom, viral RNA was recovered from faecal samples. However, this was the only study identified during literature searches specifically investigating expression of AI viral receptors in the human GI tract. While epidemiological evidence does not indicate that the GI tract is a common route for human infection by AI viruses, these results highlight that it remains a possibility.
This risk assessment was produced using a multidimensional model of risk which includes the probability of an adverse effect occurring alongside the detriment (harm or damage) associated with the severity of the microbiological hazard. The uncertainties associated with these categories and additional uncertainties are also considered (see Appendix 2; ACMSF, 2020).
As included in the risk question, the risk characterisations below are considered on a UK population basis. For some products or activities (like consuming game birds or processing game birds), the UK subpopulation of people engaging in that activity are considered as opposed to the entire UK population. This is based on the evidence that a small percentage of the UK population engages in these activities.
5.1 Frequency of occurrence of AI from commercial poultry products
Data presented in section 4.1 indicates that HPAI-infected commercial flocks of chicken and turkeys would likely be identified before being slaughtered and that LPAI-infected flocks would not likely have the virus present in the muscle tissue or organs. This means that contaminated meat is unlikely to reach the consumer. The situation may be different for commercially reared duck and geese, as they are less likely to exhibit clinical signs of infection.
While the consumption data indicates that chicken and turkey are the most commonly consumed poultry products in the UK, they are also very unlikely to be eaten pink. However, duck and geese are more likely to be eaten less than thoroughly cooked. Research demonstrates that AI viruses are heat-labile, so thorough cooking would inactivate any virus present. Additionally, proper hygienic handling of raw product would reduce the amount of any virus encountered by consumers from cross-contamination. Finally, there is the species-barrier present for infection given the receptors located in the upper human GI tract are not conducive to infection with AI viruses.
Given the above differences, the commercial poultry group was split to consider chicken and turkeys separately from duck and geese. The frequency of occurrence for the UK population of acquiring AI from the handling and consuming of commercial chicken and turkey is negligible (so rare that it does not merit to be considered). This is supported by the lack of evidence of any previous foodborne transmission of this virus from cooked poultry products. The level of uncertainty related to this is low, as there are several research studies demonstrating the inactivation of AI from cooking and disinfection.
The frequency of occurrence for the UK population of acquiring AI from the handling and consuming of commercial duck and geese is very low (very rare but cannot be excluded) with medium uncertainty. This uncertainty is because less research has focused on ducks and geese compared to other poultry.
5.2 Frequency of occurrence of AI from game birds
Since UK AI surveillance in wild birds relies on testing of identified dead birds, it is difficult to understand the infection dynamics in the game bird population. Additionally, game birds can have very different clinical signs, with species like pheasants likely to be ill enough to not be available for shooting while species like ducks might not show any signs of infection. Fewer people in the UK consume game birds, but the meat is much more likely to be eaten pink compared to chicken or turkey. Since backyard poultry would experience conditions more similar to game birds than commercial poultry, the risk from handling and consuming meat from backyard poultry is considered similar to that presented here for handling and consuming game birds. Any virus present in either game bird meat or meat from backyard poultry would be as susceptible to disinfection and cooking as was discussed for commercial poultry.
The population considered for these products is UK consumers of game birds or backyard poultry as opposed to the UK population in general. The frequency of occurrence of acquiring avian influenza virus for this population of consumers from handling or consuming these products is very low (very rare but cannot be excluded). The level of uncertainty related to this is medium as the game bird industry is less well understood compared to commercial poultry. A lack of government knowledge on the structure and integration of the game bird industry, including the behaviour of game birds in the wild has been highlighted in previous reports (Defra, 2017b). Closer liaison with the industry and individual businesses has been recommended to gain a better understanding of this sector. Furthermore, the variety of birds that may be included in “game birds” make it difficult to generalise how infections with AI viruses will progress across all the different species.
5.2.1 Frequency of occurrence of AI from home processing of birds
This risk assessment also considered the risk of acquiring AI from home processing of birds, which might include infection via inhalation during the steps of defeathering, butchering, etc. The home processing of backyard poultry is also included here. Some game birds, such as ducks and geese, that are natural reservoirs for AI may have virus present in various tissues without clear clinical signs in the bird, possibly leading to infected birds being processed for consumption. In this situation, infection could occur either by the respiratory route from inhaling droplets aerosolised during processing or by the oral route from cross-contamination.
Given the difference in systemic infection in birds between HPAI and LPAI, these were considered separately. The population under consideration here is individuals in the UK that process birds at home. Since HPAI can be present in the feathers and blood of infected birds, the frequency of occurrence of acquiring avian influenza from processing birds at home is low (rare but does occur). This takes into account the low number of AI human infections reported worldwide during outbreaks despite the huge number of birds infected. During LPAI infections, the virus is not distributed in as many tissues throughout the bird, meaning the frequency of occurrence of acquiring AI from processing birds at home is very low (very rare but cannot be excluded). The uncertainty associated with both these risk levels is medium as data exists for the distribution of the HPAI and LPAI viruses in bird tissues but research on exposure levels to AI from home processing is limited.
5.3 Frequency of occurrence of AI from hen eggs
Evidence from both natural outbreaks and experimental infections demonstrate that AI viruses can be present in the internal contents of eggs. It can also be present on the shell. Eggs are consumed by a large portion of the UK population and, compared to the other poultry products considered in this risk assessment, are the product most likely to be consumed either raw or undercooked. AI present in or on eggs would experience the same inactivation from disinfectant or cooking as described previously. Eggs produced by backyard poultry flocks can be considered alongside those from commercial laying flocks in this risk characterisation.
Given the evidence, the frequency of occurrence to the UK population of acquiring avian influenza virus from handling and consuming hen table eggs is very low (very rare but cannot be excluded). The uncertainty associated with this is low, as there was adequate data on AI in eggs, consumption of LTTC eggs in the UK and viral inactivation from heating of egg products.
5.4 Severity of detriment for avian influenza infections in humans
As discussed in Section 3, humans can exhibit a range of symptoms when infected with AI, ranging from asymptomatic infections or mild conjunctivitis to severe respiratory illness. Considering recorded AI infections worldwide, there is a high case fatality rate of up to 50%, depending on the strain. Given this, the severity of detriment from infection of AI in humans is high (severe illness: causing life-threatening or substantial sequelae or illness of long duration) with medium uncertainty. This uncertainty is due to the lack of surveillance for AI infections in people, meaning many asymptomatic infections may go undetected. This would artificially inflate the case mortality of reported infections compared to true infection numbers.
5.5 Key uncertainties
Several factors contributed to the uncertainties associated with the different risk levels identified in this risk assessment. Key uncertainties associated with different steps of the risk pathway are outlined below.
5.5.1 Uncertainties related to frequency of occurrence of AI in poultry products
The uncertainties in each section are listed in order of priority – for example, which uncertainty would make the biggest impact to the risk assessment if they were able to be resolved.
5.5.1.1 Uncertainties related to commercial poultry
- uncertainty over the prevalence of LPAI infections in commercial poultry
- uncertainty about the potential presence of LPAI in meat/muscle tissue of commercial poultry since evidence is only from experimental studies and not natural outbreaks
- uncertainty about cross-contamination of carcasses with LPAI during slaughter
5.5.1.1.1 Uncertainties related to backyard poultry
- uncertainty of the size of the smallholder community flocks and backyard poultry population
- uncertainty over the amount of backyard poultry products produced that are consumed; including eggs and meat
- uncertainty over understanding and/or compliance of owners of backyard poultry for introducing biosecurity measures when in protected zones or their ability to recognise clinical signs of AI in their backyard flock
5.5.1.2 Uncertainties related to game birds
- uncertainty over the number of game birds shot each year and consumed through unregulated routes.
- uncertainty over potential for cross-contamination, including from faeces, from home processing of birds.
- uncertainty over the likelihood of transmission from the defeathering process, particularly if carried out in a domestic setting without appropriate PPE or ventilation.
- prevalence of AI in game birds
- variability of clinical signs caused by different strains in wild aquatic birds, such as ducks and geese.
5.5.1.3 Uncertainties related to eggs
- uncertainty around the chance HPAI-contaminated eggs would be sent for processing in the time between flock infection and detection
- uncertainty around the ability to detect LPAI infection in laying flock
- uncertainty over the presence of LPAI on eggshell or inside egg
5.5.1.4 Uncertainties related to consumer behaviour
- uncertainty around cross-contamination when preparing poultry in domestic settings.
- uncertainty around different strains and temperature inactivation during cooking
5.5.2 Uncertainties related to severity of detriment of AI infection in humans
- uncertainty about the true number of human infections given asymptomatic or mild cases due to limited surveillance in humans
- uncertainty about the severity of illness associated with AI since different strains may have different pathogenic potential in humans and the variability in response to infection due to human genetic factors.
5.5.3 Additional Uncertainties
While the uncertainties related to the frequency of occurrence of AI in humans and the severity of detriment are due to either a lack of data or variability in the population, there are some additional uncertainties around the ability of AI to cause infection from food through the oral route. This is due to the lack of epidemiological evidence associated with AI cases from food, the potential infectious dose required for infections via the oral route and the ability of the AI virus to infect tissue from receptors available along the human gastrointestinal tract. Another additional uncertainty is around the effect mutations may have on the ability of the virus to transmit between species or cause infections in humans from consumption of contaminated food.
5.6 Recommendations for future research
Additional research into some of the data gaps highlighted in Section 5.5 could help reduce the uncertainty associated with the risk characterisation of this assessment. Some suggestions are below.
5.6.1 Research related to commercial poultry
- surveillance studies to better estimate LPAI prevalence in commercial flocks
- characterisation of LPAI distribution within tissues from naturally infected birds
- quantitation of levels of infectious virus in the meat and eggs of infected birds
5.6.2 Research related to game birds
Survey of game birds to determine numbers and species of birds hunted each season, including how many are for personal use or for sale through an AGHE.
Sampling study looking at prevalence of AI in game bird populations (either in the wild or during rearing).
Thank you to the following for discussions and/or providing data:
- The British Association for Shooting and Conservation
- Ashley Banyard (APHA)
- Mark Jackson (Egg Marketing Inspectorate, APHA)
- Emma French and Rhoda Aminu (FSA)
- Lucy King (FSA)
Thank you to the following for providing comments on draft versions of this report:
- Johanna Jackson (FSA)
- Daniel Lloyd (FSA)
- Niall Grieve (FSA)
- Amie Adkin (FSA)
- Rachel Taylor (APHA)
- Marco Falchieri (APHA)
- Abd El-Hack, M.E., El-Saadony, Mohamed.T., Alqhtani, A.H., Swelum, A.A., Salem, H.M., Elbestawy, Ahmed.R., Noreldin, A.E., Babalghith, A.O., Khafaga, A.F., Hassan, M.I., El-Tarabily, K.A., 2022. The relationship among avian influenza, gut microbiota and chicken immunity: an updated overview. Poult. Sci. 101, 102021. https://doi.org/10.1016/j.psj.2022.102021
- ACMSF, 2020. Fixed-term group on multidimensional representation of risks.
- ACMSF, 2015. Assessment of the risk of avian influenza viruses via the food chain.
- Air Products, 2018. Your in-depth guide to modified atmosphere packaging.
- APHA, 2023. Confirmed findings of influenza of avian origin in non-avian wildlife [WWW Document]. GOV.UK. URL (accessed 2.8.23).
- APHA, 2022a. Rapid Risk Assessment for spread of Highly Pathogenic Avian Influenza (HPAI) H5N1 from wild birds to poultry from the shooting of wild waterfowl and wild game (including formerly captive) birds.
- APHA, 2022b. Bird flu (avian influenza): cases in wild birds [WWW Document]. GOV.UK. URL (accessed 12.16.22).
- APHA, 2018. Guidance on Legislation Covering the Marketing of Eggs.
- Barrow, P., Nair, V., Baigent, S., Atterbury, R., Clark, M., 2021. Poultry Health: A Guide for Professionals. CABI.
- BASC, 2022. Wildfowling. Br. Assoc. Shoot. Conserv. (accessed 12.12.22).
- BASC, Quarry Species & Shooting Seasons. Quarry species & shooting seasons. Br. Assoc. Shoot. Conserv. URL (accessed 12.16.22).
- Bauer, N., Dearfield, K., Dennis, S., Disney, W.T., Forsythe, K., Latimer, H.E., Malladi, S., Potter, M., Ramirez, G., 2010. Interagency Risk Assessment for the Public Health Impact of Highly Pathogenic Avian Influenza Virus in Poultry, Shell Eggs, and Egg Products 164.
- Bean, W.J., Kawaoka, Y., Wood, J.M., Pearson, J.E., Webster, R.G., 1985. Characterization of virulent and avirulent A/chicken/Pennsylvania/83 influenza A viruses: potential role of defective interfering RNAs in nature. J. Virol. 54, 151–160. https://doi.org/10.1128/JVI.54.1.151-160.1985
- Beato, M.S., Mancin, M., Bertoli, E., Buratin, A., Terregino, C., Capua, I., 2012. Infectivity of H7 LP and HP influenza viruses at different temperatures and pH and persistence of H7 HP virus in poultry meat at refrigeration temperature. Virology 433, 522–527. https://doi.org/10.1016/j.virol.2012.08.009
- Beauclercq, S., Mignon-Grasteau, S., Petit, A., Berger, Q., Lefèvre, A., Métayer-Coustard, S., Tesseraud, S., Emond, P., Berri, C., Le Bihan-Duval, E., 2022. A Divergent Selection on Breast Meat Ultimate pH, a Key Factor for Chicken Meat Quality, is Associated With Different Circulating Lipid Profiles. Front. Physiol. 13.
- Bergervoet, S.A., Germeraad, E.A., Alders, M., Roose, M.M., Engelsma, M.Y., Heutink, R., Bouwstra, R., Fouchier, R.A.M., Beerens, N., 2019. Susceptibility of Chickens to Low Pathogenic Avian Influenza (LPAI) Viruses of Wild Bird– and Poultry–Associated Subtypes. Viruses 11, 1010. https://doi.org/10.3390/v11111010
- Bertran, K., Dolz, R., Majó, N., 2014. Pathobiology of avian influenza virus infection in minor gallinaceous species: a review. Avian Pathol. 43, 9–25. https://doi.org/10.1080/03079457.2013.876529
- Bertran, K., Lee, D.-H., Criado, M.F., Smith, D., Swayne, D.E., Pantin-Jackwood, M.J., 2018. Pathobiology of Tennessee 2017 H7N9 low and high pathogenicity avian influenza viruses in commercial broiler breeders and specific pathogen free layer chickens. Vet. Res. 49, 82. https://doi.org/10.1186/s13567-018-0576-0
- Bertran, K., Pérez-Ramírez, E., Busquets, N., Dolz, R., Ramis, A., Darji, A., Abad, F.X., Valle, R., Chaves, A., Vergara-Alert, J., Barral, M., Höfle, U., Majó, N., 2011. Pathogenesis and transmissibility of highly (H7N1) and low (H7N9) pathogenic avian influenza virus infection in red-legged partridge (Alectoris rufa). Vet. Res. 42, 24. https://doi.org/10.1186/1297-9716-42-24
- Bertran, K., Swayne, D.E., 2014. High doses of highly pathogenic avian influenza virus in chicken meat are required to infect ferrets. Vet. Res. 45, 60. https://doi.org/10.1186/1297-9716-45-60
- Bird flu (avian influenza) movement licences, 2022. Bird flu (avian influenza) movement licences [WWW Document]. GOV.UK. (accessed 11.29.22).
- Bordes, L., Vreman, S., Heutink, R., Roose, M., Venema, S., Pritz-Verschuren, S.B.E., Rijks, J.M., Gonzales, J.L., Germeraad, E.A., Engelsma, M., Beerens, N., 2023. Highly Pathogenic Avian Influenza H5N1 Virus Infections in Wild Red Foxes (Vulpes vulpes) Show Neurotropism and Adaptive Virus Mutations. Microbiol. Spectr. 11, e02867-22. https://doi.org/10.1128/spectrum.02867-22
- Bosch, A., Gkogka, E., Le Guyader, F.S., Loisy-Hamon, F., Lee, A., van Lieshout, L., Marthi, B., Myrmel, M., Sansom, A., Schultz, A.C., Winkler, A., Zuber, S., Phister, T., 2018. Foodborne viruses: Detection, risk assessment, and control options in food processing. Int. J. Food Microbiol. 285, 110–128. https://doi.org/10.1016/j.ijfoodmicro.2018.06.001
- British Lion Eggs, 2022. UK Egg Industry Data | Official Egg Info [WWW Document]. URL https://www.egginfo.co.uk/egg-facts-and-figures/industry-information/da… (accessed 11.24.22).
- British Lion Eggs, n.d. British Lion Eggs | What Does the Lion Stamp Mean | Egg Info [WWW Document]. (accessed 12.28.22a).
- British Lion Eggs, n.d. Egg Codes | Egg Facts | Egg Info [WWW Document]. (accessed 1.10.23b).
- Brookes, S.M., Mansfield, K.L., Reid, S.M., Coward, V., Warren, C., Seekings, J., Brough, T., Gray, D., Núñez, A., Brown, I.H., 2022. Incursion of H5N8 high pathogenicity avian influenza virus (HPAIV) into gamebirds in England. Epidemiol. Infect. 150, e51. https://doi.org/10.1017/S0950268821002740
- Brown, J.D., Goekjian, G., Poulson, R., Valeika, S., Stallknecht, D.E., 2009. Avian influenza virus in water: infectivity is dependent on pH, salinity and temperature. Vet. Microbiol. 136, 20–26. https://doi.org/10.1016/j.vetmic.2008.10.027
- Busquets, N., Abad, F.X., Alba, A., Dolz, R., Allepuz, A., Rivas, R., Ramis, A., Darji, A., Majó, N. 2010, 2010. Persistence of highly pathogenic avian influenza virus (H7N1) in infected chickens: feather as a suitable sample for diagnosis. J. Gen. Virol. 91, 2307–2313. https://doi.org/10.1099/vir.0.021592-0
- Byrne, A.M.P., Reid, S.M., Seekings, A.H., Núñez, A., Obeso Prieto, A.B., Ridout, S., Warren, C.J., Puranik, A., Ceeraz, V., Essen, S., Slomka, M.J., Banks, J., Brown, I.H., Brookes, S.M., 2021. H7N7 Avian Influenza Virus Mutation from Low to High Pathogenicity on a Layer Chicken Farm in the UK. Viruses 13, 259. https://doi.org/10.3390/v13020259
- Cappucci, D.T., Johnson, D.C., Brugh, M., Smith, T.M., Jackson, C.F., Pearson, J.E., Senne, D.A., 1985. Isolation of Avian Influenza Virus (Subtype H5N2) from Chicken Eggs during a Natural Outbreak. Avian Dis. 29, 1195. https://doi.org/10.2307/1590473
- CDC, 2023. H5N1 Update: Two Human H5N1 Cases in Cambodia [WWW Document]. Cent. Dis. Control Prev. URL (accessed 3.1.23).
- CDC, 2022a. Prevention and Antiviral Treatment of Bird Flu Viruses in People | Avian Influenza (Flu) [WWW Document]. (accessed 12.16.22).
- CDC, 2022b. Influenza Type A Viruses [WWW Document]. Cent. Dis. Control Prev. (accessed 1.6.23).
- CDC, 2022c. Reported Human Infections with Avian Influenza A Viruses [WWW Document]. (accessed 1.5.23).
- CDC, 2022d. Avian Influenza A Virus Infections in Humans [WWW Document]. Cent. Dis. Control Prev. (accessed 12.16.22).
- Center for Food Security and Public Health, 2022. Avian Influenza.
- Chmielewski, R.A., Beck, J.R., Swayne, D.E., 2013. Evaluation of the U.S. Department of Agriculture’s Egg Pasteurization Processes on the Inactivation of High-Pathogenicity Avian Influenza Virus and Velogenic Newcastle Disease Virus in Processed Egg Products. J. Food Prot. 76, 640–645. https://doi.org/10.4315/0362-028X.JFP-12-369
- Dai, M., Yan, N., Huang, Y., Zhao, L., Liao, M., 2022. Survivability of highly pathogenic avian influenza virus on raw chicken meat in different environmental conditions. Lancet Microbe 3, e92. https://doi.org/10.1016/S2666-5247(21)00333-5
- de Graaf, M., Fouchier, R.A.M., 2014. Role of receptor binding specificity in influenza A virus transmission and pathogenesis. EMBO J. 33, 823–841. https://doi.org/10.1002/embj.201387442
- De Nardi, M., Hill, A., von Dobschuetz, S., Munoz, O., Kosmider, R., Dewe, T., Harris, K., Freidl, G., Stevens, K., van der Meulen, K., Stäerk, K. d. c., Breed, A., Meijer, A., Koopmans, M., Havelaar, A., van der Werf, S., Banks, J., Wieland, B., van Reeth, K., Dauphin, G., Capua, I., Consortium, the F., 2014. Development of a risk assessment methodological framework for potentially pandemic influenza strains (FLURISK). EFSA Support. Publ. 11, 571E. https://doi.org/10.2903/sp.efsa.2014.EN-571
- de Wit, J., Fabri, T., Hoogkamer, A., 2004. Survival of Avian Influenza Virus on Eggs. Animal Health Service. Sector Research and Development Poultry Health.
- Defra, 2023a. Highly pathogenic avian influenza (HPAI) in the UK and Europe.
- Defra, 2023b. Latest poultry and poultry meat statistics [WWW Document]. GOV.UK. (accessed 2.9.23).
- Defra, 2022a. Bird flu: rules in disease control and prevention zones in England [WWW Document]. GOV.UK. (accessed 1.9.23).
- Defra, 2022b. Monthly statistics on the activity of UK hatcheries and UK poultry slaughterhouses (data for October 2022) [WWW Document]. GOV.UK. (accessed 11.21.22).
- Defra, 2021. Avian influenza (bird flu): epidemiology reports [WWW Document]. GOV.UK. (accessed 1.5.23).
- Defra, 2017a. Avian influenza (bird flu) controls: Rules on meat produced from poultry and farmed game birds originating in Protection Zone(s) [WWW Document]. (accessed 12.2.22).
- Defra, 2017b. Highly Pathogenic Avian Influenza H5N8: Lessons identified from the December 2016 to June 2017 outbreak 31.
- Defra, 2011. Poultrymeat Quality Guide.
- DHSC, 2013. Diet and nutrition survey of infants and young children, 2011 [WWW Document]. GOV.UK. (accessed 2.7.22).
- EFSA, 2023. Avian influenza overview September – December 2022. EFSA J. 21, e07786. https://doi.org/10.2903/j.efsa.2023.7786
- EFSA, 2014. Scientific Opinion on the public health risks of table eggs due to deterioration and development of pathogens | EFSA [WWW Document]. (accessed 12.28.22).
- EFSA, 2006. Scientific Report of the Scientific Panel on Biological Hazards on “Food as a possible source of infection with highly pathogenic avian influenza viruses for humans and other mammals.” EFSA J. https://doi.org/10.2903/j.efsa.2006.74r
- EFSA, E.F.S., European Centre for Disease Prevention and Control, Influenza, E.U.R.L. for A., Adlhoch, C., Fusaro, A., Gonzales, J.L., Kuiken, T., Marangon, S., Niqueux, É., Staubach, C., Terregino, C., Aznar, I., Guajardo, I.M., Baldinelli, F., 2022. Avian influenza overview March – June 2022. EFSA J. 20, e07415. https://doi.org/10.2903/j.efsa.2022.7415
- EFSA, Gonzales, J.L., Roberts, H., Smietanka, K., Baldinelli, F., Ortiz‐Pelaez, A., Verdonck, F., 2018. Assessment of low pathogenic avian influenza virus transmission via raw poultry meat and raw table eggs. EFSA J. 16. https://doi.org/10.2903/j.efsa.2018.5431
- Ejaz, R., Ahmed, Z., Siddique, N., Naeem, K., 2007. Chicken Meat as a Source of Avian Influenza Virus Persistence and Dissemination. Int. J. Poult. Sci. 6, 871–874. https://doi.org/10.3923/ijps.2007.871.874
- EXD249(HPAI)(EW), 2022. General licence for the movement of poultry meat from poultry originating in a protection zone or originating from an area that subsequently becomes a protection zone (EXD249(HPAI)(EW)) [WWW Document]. GOV.UK. (accessed 11.29.22).
- Fernandes, R.T.V., Arruda, A.M.V., Melo, A.S., Marinho, J.B.M., Fernandes, R.T.V., Figueiredo, L.C., 2016. Chemical Composition and pH of the Meat of Broilers Submitted to Pre-Slaughter Heat Stress. J. Anim. Behav. Biometeorol. 4, 93–95. https://doi.org/10.14269/2318-1265/jabb.v4n4p93-95
- Fouchier, R.A.M., Schneeberger, P.M., Rozendaal, F.W., Broekman, J.M., Kemink, S.A.G., Munster, V., Kuiken, T., Rimmelzwaan, G.F., Schutten, M., van Doornum, G.J.J., Koch, G., Bosman, A., Koopmans, M., Osterhaus, A.D.M.E., 2004. Avian influenza A virus (H7N7) associated with human conjunctivitis and a fatal case of acute respiratory distress syndrome. Proc. Natl. Acad. Sci. U. S. A. 101, 1356–1361. https://doi.org/10.1073/pnas.0308352100
- Freath, L., Bacigalupo, S., Bronw, I., Banyard, A., Pacey, A., Gale, P., Perrin, L., 2022. Highly pathogenic avian influenza (HPAI) in the UK and Europe: 14 October 2022.
- FSA, 2022a. Home food fact checker [WWW Document]. Food Stand. Agency. (accessed 12.28.22).
- FSA, 2022b. Manual for Offical Controls. Chapter 6 Notifiable Diseases.
- FSA, 2021. Food and You 2 - Wave 2 [WWW Document]. Food Stand. Agency. https://doi.org/10.46756/sci.fsa.dws750
- FSA, F., 2015. Wild game guidance [WWW Document]. Food Stand. Agency. (accessed 12.19.22).
- Gaide, N., Foret-Lucas, C., Figueroa, T., Vergne, T., Lucas, M.-N., Robertet, L., Souvestre, M., Croville, G., Le Loc’h, G., Delverdier, M., Guérin, J.-L., 2021. Viral tropism and detection of clade 2.3.4.4b H5N8 highly pathogenic avian influenza viruses in feathers of ducks and geese. Sci. Rep. 11, 5928. https://doi.org/10.1038/s41598-021-85109-5
- GFA | Game Farming in The UK [WWW Document], n.d. (accessed 12.16.22).
- GFA, G., 2021. Standing advice on bird flu and gamebirds [WWW Document]. (accessed 12.19.22).
- Golden, N.J., Schlosser, W.D., Ebel, E.D., 2009. Risk Assessment to Estimate the Probability of a Chicken Flock Infected with H5N1 Highly Pathogenic Avian Influenza Virus Reaching Slaughter Undetected. Foodborne Pathog. Dis. 6, 827–835. https://doi.org/10.1089/fpd.2008.0253
- GOV.UK, 2022a. Poultry (including game birds): registration rules and forms [WWW Document]. GOV.UK. (accessed 1.9.23).
- GOV.UK, 2022b. Bird flu: rules if you keep game birds [WWW Document]. GOV.UK. (accessed 12.19.22).
- GOV.UK, 2022c. Avian influenza: Housing order to be introduced across England [WWW Document]. GOV.UK. (accessed 12.28.22).
- Guan, J., Chan, M., Brooks, B.W., Rohonczy, E., 2015. Enhanced inactivation of avian influenza virus at −20°C by disinfectants supplemented with calcium chloride or other antifreeze agents. Can. J. Vet. Res. 79, 347–350.
- Guidance on Temperature Control in Food Premises, n.d.
- Harder, Buda, S., Hengel, H., Beer, M., Mettenleiter, T.C., 2016. Poultry food products—a source of avian influenza virus transmission to humans? Clin. Microbiol. Infect. 22, 141–146. https://doi.org/10.1016/j.cmi.2015.11.015
- Harris, K.A., Freidl, G.S., Munoz, O.S., von Dobschuetz, S., De Nardi, M., Wieland, B., Koopmans, M.P.G., Stärk, K.D.C., van Reeth, K., Dauphin, G., Meijer, A., de Bruin, E., Capua, I., Hill, A.A., Kosmider, R., Banks, J., Stevens, K., van der Werf, S., Enouf, V., van der Meulen, K., Brown, I.H., Alexander, D.J., Breed, A.C., the FLURISK Consortium, 2017. Epidemiological Risk Factors for Animal Influenza A Viruses Overcoming Species Barriers. EcoHealth 14, 342–360. https://doi.org/10.1007/s10393-017-1244-y
- Hauck, R., Crossley, B., Rejmanek, D., Zhou, H., Gallardo, R.A., 2017. Persistence of Highly Pathogenic and Low Pathogenic Avian Influenza Viruses in Footbaths and Poultry Manure. Avian Dis. 61, 64–69.
- HSE, H., 2018. Avoiding the risk of infection when working with poultry that is suspected of having H5 or H7 notifiable avian influenza.
- Hutchinson, E.C., 2018. Influenza Virus. Trends Microbiol. 26, 809–810. https://doi.org/10.1016/j.tim.2018.05.013
- Kabir, Md.H., Miyaoka, Y., Hasan, Md.A., Yamaguchi, M., Shoham, D., Murakami, H., Takehara, K., 2021. Synergistic effects of quaternary ammonium compounds and food additive grade calcium hydroxide on microbicidal activities at low temperatures. J. Vet. Med. Sci. 83, 1820–1825. https://doi.org/10.1292/jvms.21-0275
- Karunakaran, A.C., Murugkar, H.V., Kumar, M., Nagarajan, S., Tosh, C., Pathak, A., Mekhemadhom Rajendrakumar, A., Agarwal, R.K., 2019. Survivability of highly pathogenic avian influenza virus (H5N1) in naturally preened duck feathers at different temperatures. Transbound. Emerg. Dis. 66, 1306–1313. https://doi.org/10.1111/tbed.13148
- Keawcharoen, J., Oraveerakul, K., Kuiken, T., Fouchier, R.A.M., Amonsin, A., Payungporn, S., Noppornpanth, S., Wattanodorn, S., Theamboonlers, A., Tantilertcharoen, R., Pattanarangsan, R., Arya, N., Ratanakorn, P., Osterhaus, A.D.M.E., Poovorawan, Y., 2004. Avian Influenza H5N1 in Tigers and Leopards. Emerg. Infect. Dis. 10, 2189–2191. https://doi.org/10.3201/eid1012.040759
- Keeler, S.P., Dalton, M.S., Cressler, A.M., Berghaus, R.D., Stallknecht, D.E., 2014. Abiotic factors affecting the persistence of avian influenza virus in surface waters of waterfowl habitats. Appl. Environ. Microbiol. 80, 2910–2917. https://doi.org/10.1128/AEM.03790-13
- Kuiken, T., Rimmelzwaan, G., van Riel, D., van Amerongen, G., Baars, M., Fouchier, R., Osterhaus, A., 2004. Avian H5N1 Influenza in Cats. Science 306, 241–241. https://doi.org/10.1126/science.1102287
- Limsuwat, N., Suptawiwat, O., Boonarkart, C., Puthavathana, P., Auewarakul, P., Wiriyarat, W., 2014. Susceptibility of human and avian influenza viruses to human and chicken saliva. J. Med. Virol. 86, 872–878. https://doi.org/10.1002/jmv.23751
- Limsuwat, N., Suptawiwat, O., Boonarkart, C., Puthavathana, P., Wiriyarat, W., Auewarakul, P., 2016. Sialic acid content in human saliva and anti-influenza activity against human and avian influenza viruses. Arch. Virol. 161, 649–656. https://doi.org/10.1007/s00705-015-2700-z
- Liu, W.J., Xiao, H., Dai, L., Liu, D., Chen, J., Qi, X., Bi, Y., Shi, Y., Gao, G.F., Liu, Y., 2021. Avian influenza A (H7N9) virus: from low pathogenic to highly pathogenic. Front. Med. 15, 507–527. https://doi.org/10.1007/s11684-020-0814-5
- Love Food Hate Waste, 2022. Poultry (for example, chicken and turkey) [WWW Document]. Love Food Hate Waste. (accessed 1.17.23).
- Lu, H., Dunn, P.A., Wallner-Pendleton, E.A., Henzler, D.J., Kradel, D.C., Liu, J., Shaw, D.P., Miller, P., 2004. Investigation of H7N2 Avian Influenza Outbreaks in Two Broiler Breeder Flocks in Pennsylvania, 2001–02. Avian Dis. 48, 26–33. https://doi.org/10.1637/6063
- Mallapaty, S., 2023. Girl who died of bird flu did not have widely-circulating variant. Nature. https://doi.org/10.1038/d41586-023-00585-1
- More, S., Bicout, D., Bøtner, A., Butterworth, A., Calistri, P., Depner, K., Edwards, S., Garin‐Bastuji, B., Good, M., Gortázar Schmidt, C., Michel, V., Miranda, M.A., Nielsen, S.S., Raj, M., Sihvonen, L., Spoolder, H., Thulke, H., Velarde, A., Willeberg, P., Winckler, C., Breed, A., Brouwer, A., Guillemain, M., Harder, T., Monne, I., Roberts, H., Baldinelli, F., Barrucci, F., Fabris, C., Martino, L., Mosbach‐Schulz, O., Verdonck, F., Morgado, J., Stegeman, J.A., 2017. Avian influenza. EFSA J. 15, e04991. https://doi.org/10.2903/j.efsa.2017.4991
- NADIS, N., 2016. NADIS Animal Health Skills - Avian Influenza [WWW Document]. (accessed 1.17.23).
- Nazir, J., Haumacher, R., Ike, A.C., Marschang, R.E., 2011. Persistence of Avian Influenza Viruses in Lake Sediment, Duck Feces, and Duck Meat ▿. Appl. Environ. Microbiol. 77, 4981–4985. https://doi.org/10.1128/AEM.00415-11
- Ngunjiri, J.M., Taylor, K.J.M., Ji, H., Abundo, M.C., Ghorbani, A., KC, M., Lee, C.-W., 2021. Influenza A virus infection in turkeys induces respiratory and enteric bacterial dysbiosis correlating with cytokine gene expression. PeerJ 9, e11806. https://doi.org/10.7717/peerj.11806
- NHS, 2018. Bird flu [WWW Document]. nhs.uk. (accessed 1.6.23).
- O’Brien, B., Goodridge, L., Ronholm, J., Nasheri, N., 2021. Exploring the potential of foodborne transmission of respiratory viruses. Food Microbiol. 95, 103709. https://doi.org/10.1016/j.fm.2020.103709
- Oliver, I., Roberts, J., Brown, C.S., Byrne, A.M., Mellon, D., Hansen, R.D., Banyard, A.C., James, J., Donati, M., Porter, R., Ellis, J., Cogdale, J., Lackenby, A., Chand, M., Dabrera, G., Brown, I.H., Zambon, M., 2022. A case of avian influenza A(H5N1) in England, January 2022. Euro Surveill. Bull. Eur. Sur Mal. Transm. Eur. Commun. Dis. Bull. 27, 2200061. https://doi.org/10.2807/1560-7917.ES.2022.27.5.2200061
- Ota, M., Toyofuku, C., Thammakarn, C., Sangsriratanakul, N., Yamada, M., Nakajima, K., Kitazawa, M., Hakim, H., Alam, M.S., Shoham, D., Takehara, K., 2016. Calcinated egg shell as a candidate of biosecurity enhancement material. J. Vet. Med. Sci. 78, 831–836. https://doi.org/10.1292/jvms.16-0004
- Pantin-Jackwood, M.J., Stephens, C.B., Bertran, K., Swayne, D.E., Spackman, E., 2017. The pathogenesis of H7N8 low and highly pathogenic avian influenza viruses from the United States 2016 outbreak in chickens, turkeys and mallards. PLoS ONE 12, e0177265. https://doi.org/10.1371/journal.pone.0177265
- Peng, Xiuming, Liu, F., Wu, H., Peng, Xiaorong, Xu, Y., Wang, L., Chen, B., Sun, T., Yang, F., Ji, S., Wu, N., 2018. Amino Acid Substitutions HA A150V, PA A343T, and PB2 E627K Increase the Virulence of H5N6 Influenza Virus in Mice. Front. Microbiol. 9, 453. https://doi.org/10.3389/fmicb.2018.00453
- PHE, FSA, 2020. NDNS: results from years 9 to 11 (2016 to 2017 and 2018 to 2019) [WWW Document]. GOV.UK. (accessed 2.7.22).
- PHE, FSA, 2018. NDNS: results from years 7 and 8 (combined) [WWW Document]. GOV.UK. (accessed 2.7.22).
- PHE, FSA, 2016. NDNS: results from Years 5 and 6 (combined) [WWW Document]. GOV.UK. (accessed 2.7.22).
- PHE, FSA, 2014. NDNS: results from Years 1 to 4 (combined) [WWW Document]. GOV.UK. (accessed 2.7.22).
- Pillai, S.P.S., Saif, Y.M., Lee, C.W., 2010. Detection of Influenza A Viruses in Eggs Laid by Infected Turkeys. Avian Dis. 54, 830–833.
- Post, J., Burt, D.W., Cornelissen, J.B., Broks, V., van Zoelen, D., Peeters, B., Rebel, J.M., 2012. Systemic virus distribution and host responses in brain and intestine of chickens infected with low pathogenic or high pathogenic avian influenza virus. Virol. J. 9, 61. https://doi.org/10.1186/1743-422X-9-61
- Promkuntod, N., Antarasena, C., Prommuang, Porntip, Prommuang, Praison, 2006. Isolation of Avian Influenza Virus A Subtype H5N1 from Internal Contents (Albumen and Allantoic Fluid) of Japanese Quail ( Coturnix coturnix japonica ) Eggs and Oviduct during a Natural Outbreak. Ann. N. Y. Acad. Sci. 1081, 171–173. https://doi.org/10.1196/annals.1373.020
- Qi, X., Tan, D., Wu, C., Tang, C., Li, T., Han, X., Wang, Jing, Liu, C., Li, R., Wang, Jingyu, 2016. Deterioration of eggshell quality in laying hens experimentally infected with H9N2 avian influenza virus. Vet. Res. 47, 35. https://doi.org/10.1186/s13567-016-0322-4
- Reg (EC) 852/2004, E., 2004. Regulation (EC) No 852/2004 of the european parliament and of the council of 29 April 2004 on the hygiene of foodstuffs [WWW Document]. (accessed 12.19.22).
- Reg (EC) 853/2004, E., 2004. Regulation (EC) No 853/2004 of the European Parliament and of the Council of 29 April 2004 laying down specific hygiene rules for food of animal origin [WWW Document]. (accessed 12.12.22).
- Reperant, L.A., van de Bildt, M.W.G., van Amerongen, G., Leijten, L.M.E., Watson, S., Palser, A., Kellam, P., Eissens, A.C., Frijlink, H.W., Osterhaus, A.D.M.E., Kuiken, T., 2012. Marked endotheliotropism of highly pathogenic avian influenza virus H5N1 following intestinal inoculation in cats. J. Virol. 86, 1158–1165. https://doi.org/10.1128/JVI.06375-11
- Rice, E.W., Adcock, N.J., Sivaganesan, M., Brown, J.D., Stallknecht, D.E., Swayne, D.E., 2007. Chlorine Inactivation of Highly Pathogenic Avian Influenza Virus (H5N1). Emerg. Infect. Dis. 13, 1568–1570. https://doi.org/10.3201/eid1310.070323
- Roy Chowdhury, I., Yeddula, S.G.R., Kim, S.-H., 2019. Pathogenicity and Transmissibility of North American H7 Low Pathogenic Avian Influenza Viruses in Chickens and Turkeys. Viruses 11, 163. https://doi.org/10.3390/v11020163
- Samanta, I., Bandyopadhyay, S., 2017. Infectious Diseases. Pet Bird Dis. Care 13–166. https://doi.org/10.1007/978-981-10-3674-3_2
- Shahid, M.A., Abubakar, M., Hameed, S., Hassan, S., 2009. Avian influenza virus (H5N1); effects of physico-chemical factors on its survival. Virol. J. 6, 38. https://doi.org/10.1186/1743-422X-6-38
- Shibata, A., Okamatsu, M., Sumiyoshi, R., Matsuno, K., Wang, Z.-J., Kida, H., Osaka, H., Sakoda, Y., 2018. Repeated detection of H7N9 avian influenza viruses in raw poultry meat illegally brought to Japan by international flight passengers. Virology 524, 10–17. https://doi.org/10.1016/j.virol.2018.08.001
- Shinya, K., Makino, A., Tanaka, H., Hatta, M., Watanabe, T., Le, M.Q., Imai, H., Kawaoka, Y., 2011. Systemic Dissemination of H5N1 Influenza A Viruses in Ferrets and Hamsters after Direct Intragastric Inoculation ▿. J. Virol. 85, 4673–4678. https://doi.org/10.1128/JVI.00148-11
- Shu, Y., Li, C.K., Li, Z., Gao, R., Liang, Q., Zhang, Y., Dong, L., Zhou, J., Dong, J., Wang, D., Wen, L., Wang, M., Bai, T., Li, D., Dong, X., Yu, H., Yang, W., Wang, Y., Feng, Z., McMichael, A.J., Xu, X., 2010. Avian Influenza A(H5N1) Viruses Can Directly Infect and Replicate in Human Gut Tissues. J. Infect. Dis. 201, 1173–1177. https://doi.org/10.1086/651457
- Skufca, J., Bell, L., Molino, J.C.P., Saulo, D., Lee, C.-K., Otsu, S., Co, K.C., Chiew, M., Leuangvilay, P., Patel, S., Khalakdina, A., Ieng, V., Matsui, T., Olowokure, B., 2022. An epidemiological overview of human infections with HxNy avian influenza in the Western Pacific Region, 2003–2022. West. Pac. Surveill. Response J. 13. https://doi.org/10.5365/wpsar.2022.13.4.987
- Sonthipet, S., Ruenphet, S., Takehara, K., 2018. Bactericidal and virucidal efficacies of potassium monopersulfate and its application for inactivating avian influenza virus on virus-spiked clothes. J. Vet. Med. Sci. 80, 568–573. https://doi.org/10.1292/jvms.17-0599
- Spackman, E., 2020. A Brief Introduction to Avian Influenza Virus. Methods Mol. Biol. Clifton NJ 2123, 83–92. https://doi.org/10.1007/978-1-0716-0346-8_7
- Steel, J., Lowen, A.C., Mubareka, S., Palese, P., 2009. Transmission of Influenza Virus in a Mammalian Host Is Increased by PB2 Amino Acids 627K or 627E/701N. PLOS Pathog. 5, e1000252. https://doi.org/10.1371/journal.ppat.1000252
- Swayne, D., 2022. Avian Influenza [WWW Document]. MSD Vet. Man. (accessed 1.6.23).
- Swayne, D.E., 2006. Microassay for measuring thermal inactivation of H5N1 high pathogenicity avian influenza virus in naturally infected chicken meat. Int. J. Food Microbiol. 108, 268–271. https://doi.org/10.1016/j.ijfoodmicro.2005.08.032
- Swayne, D.E., Beck, J.R., 2005. Experimental study to determine if low-pathogenicity and high-pathogenicity avian influenza viruses can be present in chicken breast and thigh meat following intranasal virus inoculation. Avian Dis. 49, 81–85. https://doi.org/10.1637/7260-081104R
- Swayne, D.E., Beck, J.R., 2004. Heat inactivation of avian influenza and Newcastle disease viruses in egg products. Avian Pathol. 33, 512–518. https://doi.org/10.1080/03079450400003692
- Swayne, D.E., Eggert, D., Beck, J.R., 2012. Reduction of high pathogenicity avian influenza virus in eggs from chickens once or twice vaccinated with an oil-emulsified inactivated H5 avian influenza vaccine. Vaccine 30, 4964–4970. https://doi.org/10.1016/j.vaccine.2012.05.041
- The Avian Influenza and Influenza of Avian Origin in Mammals (England) (No.2) Order 2006, E., 2006. The Avian Influenza and Influenza of Avian Origin in Mammals (England) (No.2) Order 2006 [WWW Document]. (accessed 12.14.22).
- The Eggs and Chicks (England) Regulations 2009 [WWW Document], n.d. (accessed 12.28.22).
- The Food Safety and Hygiene (England) Regulations 2013 [WWW Document], n.d. (accessed 3.3.23).
- Tumpey, T.M., Suarez, D.L., Perkins, L.E.L., Senne, D.A., Lee, J., Lee, Y.-J., Mo, I.-P., Sung, H.-W., Swayne, D.E., 2002. Characterization of a Highly Pathogenic H5N1 Avian Influenza A Virus Isolated from Duck Meat. J. Virol. 76, 6344–6355. https://doi.org/10.1128/JVI.76.12.6344-6355.2002
- Uchida, Y., Takemae, N., Tanikawa, T., Kanehira, K., Saito, T., 2016. Transmission of an H5N8-Subtype Highly Pathogenic Avian Influenza Virus from Infected Hens to Laid Eggs. Avian Dis. 60, 450–453. https://doi.org/10.1637/11312-110315-Reg
- UKHSA, 2023. Technical risk assessment for avian influenza (human health): influenza A H5N1 2.3.4.4b [WWW Document]. GOV.UK. (accessed 3.3.23).
- UKHSA, 2022. Investigation into the risk to human health of avian influenza (influenza A H5N1) in England: technical briefing 1.
- Vietnamese Has Bird Flu After Drinking Duck Blood [WWW Document], n.d. (accessed 1.9.23).
- Vreman, S., Bergervoet, S., Zwart, R., Stockhofe-Zurwieden, N., Beerens, N., 2022. Tissue tropism and pathology of highly pathogenic avian influenza H5N6 virus in chickens and Pekin ducks. Res. Vet. Sci. 146. https://doi.org/10.1016/j.rvsc.2022.03.010
- Wanaratana, S., Tantilertcharoen, R., Sasipreeyajan, J., Pakpinyo, S., 2010. The inactivation of avian influenza virus subtype H5N1 isolated from chickens in Thailand by chemical and physical treatments. Vet. Microbiol. 140, 43–48. https://doi.org/10.1016/j.vetmic.2009.07.008
- Wang, D., Zhu, W., Yang, L., Shu, Y., 2021. The Epidemiology, Virology, and Pathogenicity of Human Infections with Avian Influenza Viruses. Cold Spring Harb. Perspect. Med. 11, a038620. https://doi.org/10.1101/cshperspect.a038620
- WHO, 2023. Avian Influenza Weekly Update Number 878.
- WHO, 2022. Assessment of risk associated with recent influenza A(H5N1) clade 2.3.4.4b viruses.
- WHO, 2020. Influenza: Avian [WWW Document]. (accessed 12.16.22).
- WHO, 2018. Influenza (Avian and other zoonotic) [WWW Document]. (accessed 12.16.22).
- WHO, 2023, n.d.
- Wiwanitkit, V., 2007. Can avian bird flu virus pass through the eggshell? An appraisal and implications for infection control. Am. J. Infect. Control 35, 71. https://doi.org/10.1016/j.ajic.2006.08.006
- Wood, J.P., Choi, Y.W., Chappie, D.J., Rogers, J.V., Kaye, J.Z., 2010. Environmental Persistence of a Highly Pathogenic Avian Influenza (H5N1) Virus. Environ. Sci. Technol. 44, 7515–7520. https://doi.org/10.1021/es1016153
- Wu, L., Mitake, H., Kiso, M., Ito, M., Iwatsuki-Hirimoto, K., Yamayoshi, S., Lopes, T.J.S., Feng, H., Sumiyoshi, R., Shibata, A., Osaka, H., Imai, M., Watanabe, T., Kawaoka, Y., 2020. Characterization of H7N9 avian influenza viruses isolated from duck meat products. Transbound. Emerg. Dis. 67, 792–798. https://doi.org/10.1111/tbed.13398
- Yamamoto, Y., Nakamura, K., Mase, M., 2017. Survival of Highly Pathogenic Avian Influenza H5N1 Virus in Tissues Derived from Experimentally Infected Chickens. Appl. Environ. Microbiol. 83, e00604-17. https://doi.org/10.1128/AEM.00604-17
- Yamamoto, Y., Nakamura, K., Yamada, M., Mase, M., 2010. Persistence of avian influenza virus (H5N1) in feathers detached from bodies of infected domestic ducks. Appl. Environ. Microbiol. 76, 5496–5499. https://doi.org/10.1128/AEM.00563-10
- Zhao, C., Pu, J., 2022. Influence of Host Sialic Acid Receptors Structure on the Host Specificity of Influenza Viruses. Viruses 14, 2141. https://doi.org/10.3390/v14102141
- Zou, S., Guo, J., Gao, R., Dong, L., Zhou, J., Zhang, Y., Dong, J., Bo, H., Qin, K., Shu, Y., 2013. Inactivation of the novel avian influenza A (H7N9) virus under physical conditions or chemical agents treatment. Virol. J. 10, 289. https://doi.org/10.1186/1743-422X-10-289
This risk characterisation section of this risk assessment followed guidelines produced by the Advisory Committee on the Microbiological Safety of Food (ACMSF, 2020), where the frequency of occurrence and the severity of detriment are considered separately. The tables demonstrating the different levels of risk and uncertainty considered when concluding the risk characterisation are included below.
Table A2.1: A qualitative scale for the frequency of occurrence of foodborne risks
Frequency category | Interpretation |
---|---|
Negligible | So rare that it does not merit to be considered |
Very low | Very rare but cannot be excluded |
Low | Rare but does occur |
Medium | Occurs regularly |
High | Occurs very often |
Very High | Events occur almost certainly |
Table A2.2 - A qualitative scale for the severity of detriment of foodborne risks
Severity category | Interpretation |
---|---|
Negligible | No effects, or so mild they do not merit to be considered |
Low | Mild illness: not usually life-threatening, usually no sequelae, normally of short duration, symptoms are self-limiting (for example transient diarrhoea) |
Medium | Moderate illness: incapacitating but not usually life-threatening, sequelae rare, moderate duration (for example diarrhoea requiring hospitalisation) |
High | Severe illness: causing life-threatening or substantial sequelae or illness of long duration (for example chronic hepatitis) |
Table A2.3 - A qualitative scale for the level of uncertainty in food risk assessment
Uncertainty category | Interpretation |
---|---|
Low | There are solid and complete data available; strong evidence is provided in multiple references; authors report similar conclusions |
Medium | There are some but no complete data available; evidence is provided in small number of references; authors report conclusions that vary from one another |
High | There are scarce or no data; evidence is not provided in references but rather in unpublished reports or based on observations, or personal communication; authors report conclusions that vary considerably between them |
Revision log
Published: 20 June 2023
Last updated: 20 June 2024