Technological foundations of 3D and 4D printing of food
A systematic overview of the technological principles most relevant to the 3D printing of food will be presented and technical specifics of interest for the wider context of the future evolution of the technology will be highlighted. This should enable the FSA to get a baseline understanding of the technical aspects as relevant for its remit.
A systematic overview of the technological principles most relevant to the 3D printing of food will be presented and technical specifics of interest for the wider context of the future evolution of the technology will be highlighted. This should enable the FSA to get a baseline understanding of the technical aspects as relevant for its remit.
2.1 General aspects of 3D food printing technologies
Setting up a food printing process requires still considerable effort to adjust parameters and device settings to the exact food type considered, even with commercially available printers already optimised for certain food types. See figure 3 for relevant engineering considerations that are important for setting up a food printing process.
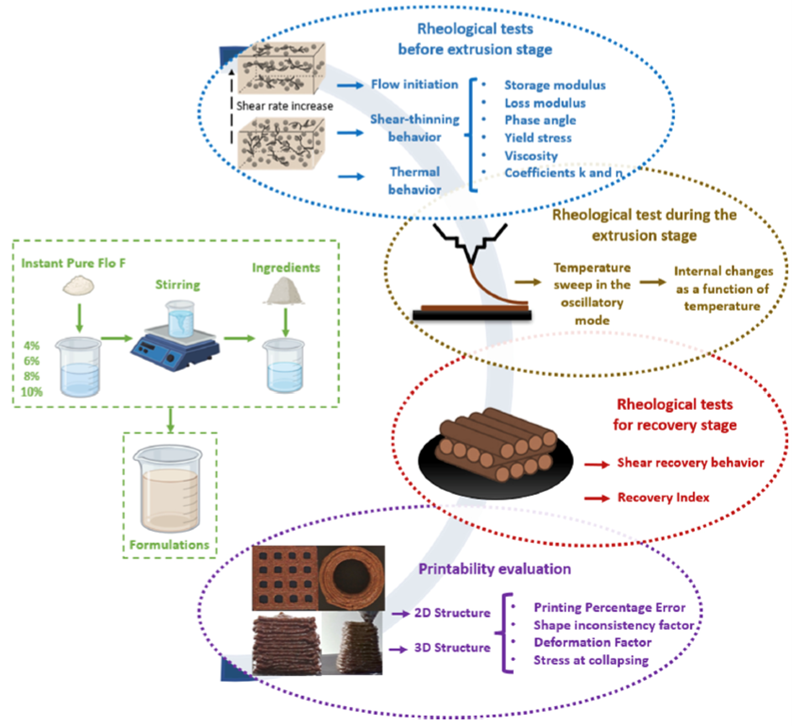
Figure 3: Workflow of engineering considerations important for printing success using an extrusion printing device. Source: Maldonado-Rosas et al., 2022
Rheological tests before extrusion stage:
Shear rate increase
Flow initiation, Shear-thinking behaviour, Thermal behaviour
- Storage modules
- Loss modulus
- Phase angle
- Yield stress
- Viscosity
- Coefficients k and n
Rheological test during the extrusion stage
Temperature sweep in the oscillatory mode
Internal changes as a function of temperature
Rheological tests for recovery stage
Shear recovery behaviour
Recovery index
Printability evaluation
2D Structure, 3D structure
- Printing percentage error
- Shape inconsistency factor
- Deformation factor
- Stress at collapsing
Choosing the best printing methodology for respective food types is crucial for achieving good results. For every ingredient type recipes for ‘food inks’ need to be optimised for the respective printer technology, depending on for example viscosity of food pastes or mesh size of powders, water content etc. (for details see section 3.4). These pre-processing steps can involve automated mixing devices that can be part of the printer mechanism or be independent of the printer, but reproducible and consistent ingredient preparation is essential for printer performance, and incorrect pre-processing can lead to printer failure. In turn, the specific device settings of the printing process need to be established empirically for each food type, for example in extrusion printing with regards to nozzle diameter, printing speed, extrusion rate, layer height and temperature, including adapting to variable external parameters such as room temperature, air humidity etc. (Isabel Diañez et al., 2022). Hence, some printer manufacturers also offer pre-prepared food inks for their printers to ensure quality of consumer printed products. Finally, many printed food items require time-critical post-processing, such as frying, baking, boiling or freezing, which need to be optimised for the food items in question.
Irrespective of the specific printing technology, all current printers require a computer interface that loads and processes 3D image files to communicate user inputs and control commands to the mechanical control unit that drives a form of xyz motorised stage and nozzle arm and other movable parts, such as pumps and mixing screws. The printing process usually involves first model building via CAD software or by scanning a work piece sample, then conversion of the model into a Standard Triangle Language (STL) file that allows building the object layer-by-layer based on x,y,z coordinates, and subsequent robotic execution of STL file commands via G- and M-codes during the printing process creating the 3D object (Baiano, 2022; Guo et al., 2019; Nachal et al., 2019). It was generally innovations around these aspects of the 3D printing technology that have enabled user friendly interfaces and more robust hardware made specifically for food printing that helped bring down prices for devices so that currently a small range of food printers is available at different price points (see section 5.2 on available printers). In many cases some form of post-processing of the printed object is required, which is usually independent from the printer, although printer developers are working on integrated cooking systems (see section 3.2).
2.2 Types of most common printing principles
Despite the variety of 3D printing systems that exists for other materials, only a limited number have proven to be suitable for printing food, due to the specific physical and chemical properties of food ingredients. We give here a top-level overview of the most widely used printing principles as printed food is exposed to quite different technical environments as well as physical and chemical impacts depending on printer type, which may pose different risks with respect to food safety. At present the following four 3D printing principles are used for food printing, and commercialised printers exist for each of them, although they are not all available for sale. These are: Extrusion-based printing, binder jetting, ink jet printing, and selective laser or hot air sintering.
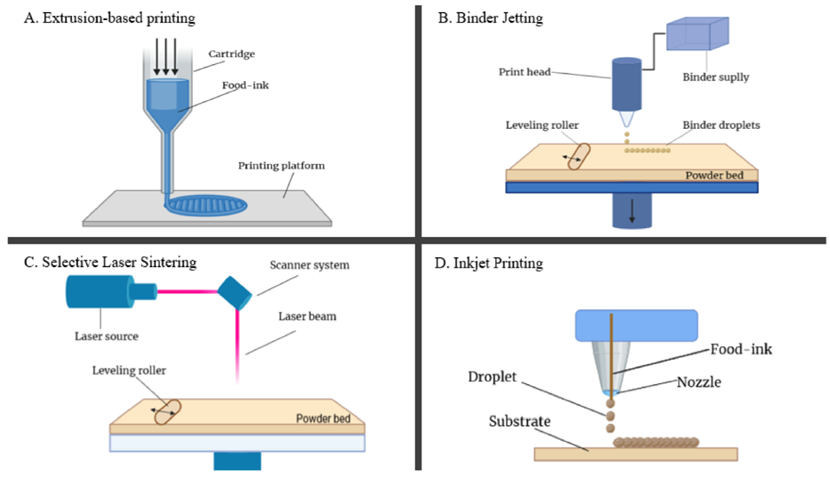
Figure 4: The most common printing principles used for 3D printing of food. Source: Varvara et al., 2021.
2.2.1 Extrusion-based printing
Extrusion printing (fig 4a) is by far the most versatile and most widely applied technique and is based on the extrusion of soft, semi-fluid food pastes, or food inks of a certain viscosity from a cartridge through a nozzle via a pump mechanism. A number of extrusion mechanisms exist but the most common are, syringe-based, air pressure-based, or screw-based.
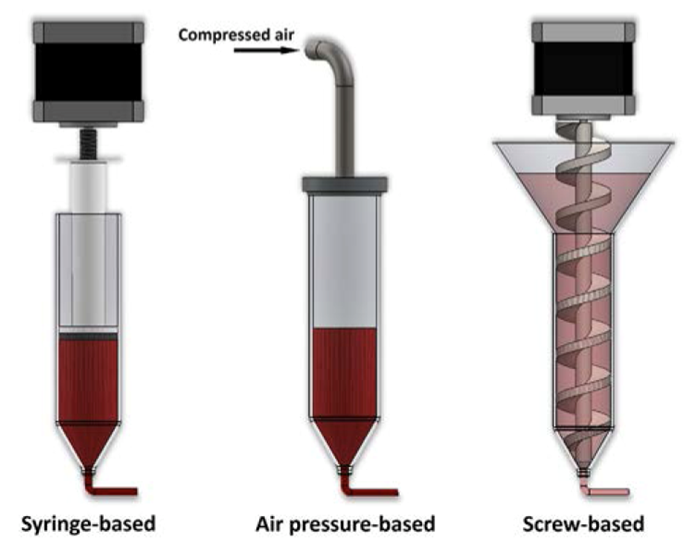
Figure 5: Most common extrusion mechanism used in extrusion printers. Source: Diañez et al., 2022
The final composition and viscosity of food ink can be achieved in some printers by a mixing and dosing system that is part of the printer device and directly delivers the ingredient mixture into the printer cartridge. This process can involve mixing powders, pastes and liquids to produce the desired food ink composition. However the majority of extrusion printers currently require the food ink being already correctly pre-prepared and just loaded into the cartridge.
In most extrusion-based printers the nozzle can be heated to provide optimal flow conditions depending on the temperature-dependent viscosity of the ingredient mixture. Such Hot Melt Extrusion (HME) applications have been used with various materials including many plastics as well as in the pharmaceuticals sector to mix ingredients for medicine formulations (Liu et al., 2017). The 3D structure is deposited onto the printing platform by moving the nozzle in the x,y dimensions and the platform is lowered along the z-axis as the object is deposited layer by layer (or, in some printers the nozzle is moved upwards along the z-axis). With this method for example pre-tempered chocolate, seafood processing waste and meat puree, cheese, different types of dough as well as potato starch-based food items can be printed successfully as well as some fruit and vegetable-based food inks. Several publications have established various ways of optimising extrusion printing parameters for these food items through specific temperature and nozzle control settings and various food additives, such as emulsifiers and thickeners, and a wide range of food standards compliant hydrocolloids (e.g. guar gum, xanthan gum, starch, pectin, gelatine etc.) to achieve a good trade-off between layer bonding, structural stability and reasonable printing speed (Liu & Zhang, 2019; Wilms et al., 2021). The choice of additives for optimising the printing process needs to consider any post-processing steps such as baking, cooking, boiling, microwaving, or frying, as additives affect final consistency and shape of the food product.
Extrusion-based printing is so far the most versatile printing process with the greatest potential for printing also healthy foods as it allows printing of fresh ingredients, such as fruit and vegetables and various ‘functional’ ingredients after addition of the right hydrocolloids, as in principle temperatures can be kept low enough to preserve molecular integrity and nutritional value of such ingredients. Extrusion-based printing is also the method of choice for any personalisation of printed foods due to the ability to control ingredient composition with various precise mixing mechanisms (Ma & Zhang, 2022). Successful printing of meat, plant-based meat and seafood paste (Surimi) and cultured (in-vitro) meat as well as cultured seafood have been reported. For these latter applications it was recommended to keep temperatures below 4C° throughout all printing steps in order to prevent bacterial growth, although no experiments have been carried out to establish specific temperature conditions (Dick et al., 2019; New Food, 2022).
The engineering parameters of extrusion food printing have been studied in great detail over the past decade. For example, a range of extrusion pressures for certain nozzle diameters appears now established for specific food pastes, such as for soft food pastes (e.g. fish, beet puree, egg white foam etc.) with pressures between 20-50kPa, for stiffer pastes (e.g. Marmite) 100-170kPa, or for thicker multi-ingredient protein- and fibre-rich pastes with low water content in the range of 300-600kPa. Also, the engineering parameters of nozzle diameter, flow rate and printing speed are well understood for many food types. Generally, a good trade-off between printing precision, shape stability, and printing speed can be achieved by calculating physical parameters of the material properties of the food inks, such as storage and loss modulus and yield stress, and applying standard engineering equations. However, printing speeds are still slow compared to industrial food production processes and the fastest printers (not yet available for consumer markets) can currently print around 700g of material per minute when printing simple structures (Wilms et al., 2021). Despite the versatility and relative simplicity of extrusion printing, challenges remain around structural stability and long-term printer performance due to the complexity of ingredient mixtures.
2.2.1.1 Types of extrusion-based printing
Within extrusion printing three main printing modes exist, these are non-phase-change extrusion, melting extrusion, and gel forming extrusion (Isabel Diañez et al., 2022; F. C. Godoi et al., 2019).
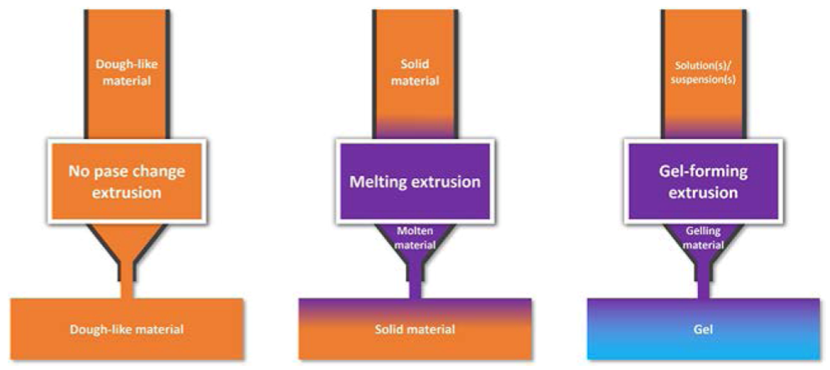
Figure 6: Types of extrusion printing. Source: Diañez et al., 2022
2.2.1.1.1 Non-phase-changing extrusion does not apply any temperature controls, such as heating or cooling, and only relies on the intrinsic rheological properties of the food material, usually at room temperature (fig 4a). This method is often used for doughs such as pasta dough and paste-like ingredients such as purees. However, food standards compliant additives, such as gums or starches are often added to improve printability.
2.2.1.1.2 Melting extrusion is based on the principle used in fused filament fabrication (FFF), which is most common for printing of plastic materials and some metals, involving a heat-induced change of phase during the printing process (usually from solid to liquid to solid). Food ingredients for melting extrusion are often highly viscous or granular pastes that need to be kept slightly above their melting point when in the extruder, but solidify quickly at lower temperatures to obtain the structural stability for the next layer to be built upon (sometimes cooling systems are used to accelerate this process) (Liu & Zhang, 2019). The most common food example for this form of extrusion printing is chocolate, as complex shapes can be generated with this method and a number of commercial chocolate printers based on this principle are, or have been, on the market (Mantihal et al., 2017), for details see section 5.2 and appendix A.
2.2.1.1.3 Gel-forming extrusion depends on the molecular properties of gel forming polymers in solution, which at specific temperatures (gel point) begin to crosslink forming a continuous network usually with large intermolecular spaces that can hold large quantities of the solvent molecules or air, hence forming hydrogels, aerogels, or oleogels. Various gels have been used in the food industry for many decades to modify rheological properties, textures and moisture content of foods and increase shelf life among other applications (Cao & Mezzenga, 2020). Their well researched properties and their role in the food industry have made gels also a prominent ingredient in 3DFP and hydrogels consisting mostly of polysaccharides and proteins are used as an edible base material that can be ‘loaded’ with various nutrient and taste enhancing ingredients as well as functional molecules. Gels are also extensively studied with respect to their role as a property modifier for improving the printing process for many otherwise difficult to print food ingredients. Many gel types can be loaded into a printer already in their set state, but ideally final gel properties are tuned at gelation point during the printing process which requires optimising temperature, printing speed, layer height and ingredient composition to achieve good results. Gel forming extrusion allows high degrees of standardisation of stable ingredient mixtures before the printing process, also allowing a high reproducibility of printed product, which is one reason why this technique has also found many applications in the biomedical and pharmaceutical sectors (Yan et al., 2018).
2.2.2 Binder jetting
Is a method that uses a binder liquid that is dispensed via the printer nozzle in the x and y axes onto a food powder bed (fig 4b). Upon coming into contact with the powder particles, the binder liquid binds these together producing a solid structure after drying. After each layer is solidified, usually by applying heat to speed up drying, a new layer of powder is applied to the powder bed via a re-coater and the process is repeated after lowering the powder bed table in the z- direction. The method allows for complex structures, reasonable speed, and stability of structures but is limited to relatively homogenous powder ingredients, such as sugar, starch, milk powder, chocolate powder, and hence mostly not suitable for nutritious food items. Binder jetting has found a niche in decorative confectionary items often using food colours, as it also allows for relatively high precision and shape complexity. One example of a binder-jetting printer outside of research laboratories is the CURRANT 3D printer, originally developed by Sugarlabs over ten years ago and possibly coming to the market soon.
2.2.3 Inkjet printing
Is not strictly a 3D method for creating layered structures but rather for dispensing mostly low viscosity food ink droplets onto the surface of food items in a patterned fashion mainly for decorative purposes or surface filling (fig 4d). The nozzle of inkjet printers does not touch the food onto which is printed. Frequently used inks are chocolate, pizza sauce or food-coloured water based food inks, such as sugar syrup. If printing onto food, the physical and chemical properties of the food surface and the ink need to be well considered and printer as well as food ink parameters matched, such that the printed pattern can achieve the desired precision and durability during and after the printing process. The method can be fast, as it is mostly not used for building 3D structures but rather for 2D designs in multiple colours. Examples are PancakeBot, Pancake Printer made by Zbot, China, or Foodjet food printing solutions, the Netherlands, which are advertised as ‘food deposition’ methods rather than food 3D printing often using large multi-nozzle arrays.
2.2.4 Selective Laser Sintering (SLS) and Hot Air Sintering (HAS)
These are powder-based methods in which the powder particles are melted and re-solidified after being heated (sintered) by either an infrared laser beam (SLS), or a focused beam of hot air (HAS) (fig 4c). After solidification of each layer on the powder bed table, a new layer of powder is applied and the powder bed table lowered along the z-axis. Like with binder jetting, only mixtures of homogenous powders with consistent mesh size of their particles, for example starches with maltodextrin, palm oil powder or sugars and various combinations of such ingredients can be used. Although the method can be fast and generate complex decorative and textural structures, as it often does not require post processing, it is not suitable for fresh or complex ingredient mixtures, as the heat of the laser/air beam are affecting molecular integrity of food ingredients, and the effect of this method on nutritional value of printed items is currently not well understood. Outside research laboratories there are currently no commercialised printers on the market using this technology.
Revision log
Published: 3 March 2023
Last updated: 5 March 2024