The Future of Animal Feed: Cellular agriculture
Recent advancements in biotechnology have opened new ways of producing livestock goods using high-quality proteins from microorganisms, including micro-algae, fungi, and bacteria, instead of using crop plants.
Similar to algae and seaweed, micro-algae are rich in fatty acids and carotenoids important to livestock from aquatic species (e.g., salmon, shrimp) to poultry and pigs and to humans who consume it mainly in the form of food supplements [Jones et al., 2020]. Fungal proteins (e.g., yeast protein) have long been used both in feeds and human foods and current research focuses on ways to optimise their production. One particularly interesting field of research looks into the potential for animal and agricultural food waste (e.g., fish, fruits) to be used as substrates for fungal protein production, therefore upgrading waste into high-value products while supplementing the livestock sector with important protein resources [Tropea et al., 2022]. Bacterial protein is another well-known alternative solution for livestock feed, which can benefit from further developments in upgrading waste or by-product substrates particularly in reducing production costs to enable it to compete with less expensive conventional feeds [Puyol et al., 2017; Ritala et al., 2017].
Cellular protein is typically produced through the ability of microorganisms to convert organic and inorganic carbon substrates into valuable protein and other nutrients, or through the use of GM/GE technologies (i.e., bioengineering) to extract such macromolecules from fermented cell cultures (e.g,. Quorn, cultivated soy-meat).
Environmental implications
Land degradation, land use change and land availability related impacts
Production of protein feeds from cellular sources is not dependent on arable land and therefore presents an effective solution to improving overall resource efficiency of the livestock sector and mitigating land-use related impacts [Nyyssölä et al., 2022]. As cellular agriculture becomes increasingly popular in the global North, it can significantly help relieve land-use pressures in high-risk forested areas of the South [Stephens & Ellis, 2020]. Cellular protein can be produced in facilities located in urban areas (e.g., labs, vertical farms), consequently freeing up agricultural land for food crops and livestock.
Greenhouse gas emissions, atmospheric pollution and fossil fuel depletion
Cellular agriculture relies less on fossil fuel than conventional protein crop production and currently operates on a mixture of fuels, consisting mainly of natural gas, coal and electricity from renewable sources; a study using US large scale cell production as a case in point indicates a fuel blend of 43% : 33% : 16% respectively [Mattick, 2018]. As a result, substituting protein crop feeds with cellular protein can help reduce fossil fuel depletion and associated GHG emissions to atmosphere. The potential for mitigation of such impacts can be increased by enabling additional usage of renewable energy sources for cellular agriculture shifts, especially since its main operations and energy requirements are for heating and artificial lighting and their automated regulation [Mattick, 2018]. Bacterial and fungal protein (e.g., methanotrophs and yeasts respectively) can reduce GHG emissions and improve resource efficiency of livestock feeds further through the utilisation of industrial wastes and residues as feedstocks, for example CH4 and CO2 emissions from oil drilling [Nyyssölä et al., 2022]. Finally, since cellular protein production can take place in urban areas and close to feed processing / manufacturing facilities and distribution hubs, it can reduce GHG atmospheric emissions and fossil fuel use associated with transportation requirements.
Nitrogen and phosphorus related impacts
Unlike conventional crops, cellular agriculture does not require synthetic fertilisers to provide the necessary nutrients for protein production. Instead, nitrogen fixating microorganisms can either recycle the reactive nitrogen available on soils and organic / inorganic substrates, or fixate atmospheric nitrogen [Helliwell & Burton, 2021; Nyyssölä et al., 2022]. These processes help reduce surpluses of nitrogen and phosphorus that could potentially lead to acidification and eutrophication of terrestrial and aquatic ecosystems.
Impacts to water quality and depletion of water resources
The water footprint of protein production from microorganisms can vary greatly depending on the type of substrate used and location of production. In cases where cellular agriculture uses plant-derived feedstuffs (e.g., sugar), total consumption of water (including requirements for climate regulation, heating and cooling of the facilities) is comparable to that of conventional crop production [Behm et al., 2022]. On the other hand, cellular agriculture generates significantly less wastewater than conventional protein crops and therefore it does not threaten water quality. Moreover, it can utilise a range of water streams such as fresh, marine and recycle wastewater, further reducing pressures on available water resources [Nyyssölä et al., 2022]. However, to best evaluate the effectiveness of this strategy to improve water quality, alternative pathways for food waste recycling should also be considered (Fig. 3) [Puyol et al., 2017].
Figure 3: Different biological technologies and pathways for wastewater treatment and recovery. Source: Puyol et al. (2017).
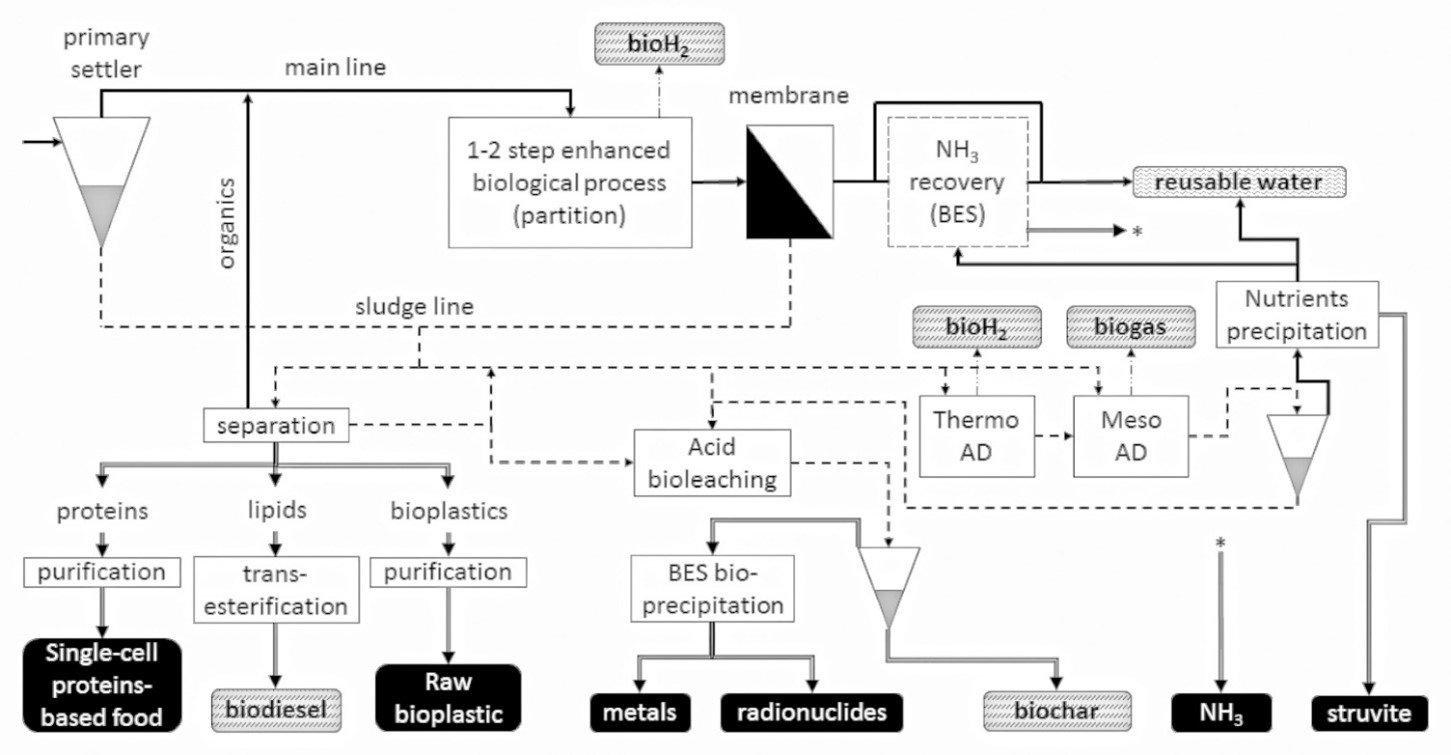
Impacts to biodiversity
Cellular agriculture contributes to the conservation of biodiversity and preservation of biodiversity hot-spot areas of the planet by reducing land requirements for protein feed production and therefore mitigating associated impacts of deforestation and habitat fragmentation / loss. However, an important consideration here is that a potential abrupt abandonment of agricultural landscapes due to a shift towards cellular agriculture may lead to a loss of grassland biodiversity and habitat fragmentation if land is mismanaged [Helliwell & Burton, 2021; Moritz et al., 2022].
Economic implications
Production and supply economics and resilience to extreme events
While more research is required to quantify the costs associated with the production of protein feeds from cellular agriculture particularly under varied production scenarios (e.g., different substrates), studies have looked into the economic of cultured meat production systems and found that they can be comparable to conventional livestock and crop production systems [Bapat et al., 2021; Eibl et al., 2021]. Advancements in biotechnology can help overcome challenges regarding the large capital investments required for production (e.g., purchasing of production-related equipment), for treatment of waste substrates to enhance biosecurity, and for sourcing of required specialised labour [Odegard & Sinke, 2021]. Such developments will make cellular protein more competitive in the feed market and help address current market uncertainties related to the willingness of feed manufacturers and livestock producers to shift away from conventional protein sources [Saavoss, 2019].
The relatively low fossil fuel requirements of cellular agriculture and ability of microorganisms to convert a range of waste streams to valuable products enables novel circular economy pathways that enhance resilience of the livestock feed production sector against fluctuations in fuel prices and prices of relevant agricultural commodities (e.g., plant-derived feedstuffs). If more and less expensive renewable energy becomes available for cellular agriculture (e.g., electricity from solar power), then its economic performance at large scales can be further improved [Eibl et al., 2021; Helliwell & Burton, 2021; Nyyssölä et al., 2022].
Considering the uncertainties and instabilities in global fuel prices and trading policies, the ability of cellular agriculture to take place in urban areas and close to distribution hubs may greatly reduce costs associated with transportation and increase feed security and availability in local livestock production systems. Finally, cellular agriculture allows for better control of the levels and quality of production, therefore providing higher consistency of representation in the market and financial security [Gasteratos, 2019].
Social implications
Nutritional value and animal growth
From a nutritional perspective, protein from cellular agriculture sources can sufficiently compare to protein levels from conventional sources. Specifically, crude protein contents from fungi (e.g., Saccharomyces cerevisiae), bacteria (e.g., Arthrospira plantensis) and micro-algae (e.g., Chlorella vulgaris) can range from 33% to 47%, 51% to 81%, and 7% to 59% respectively [Pignolet et al., 2013; Glencross et al., 2020]. Research has shown that the amino acid composition and nutritional profile of cellular protein meals that have been tested in livestock and fish feed formulations does not lead to significant differences in animal body compositions and growth [Hardy et al., 2018; Jones et al., 2020].
Animal health and welfare
Several studies have shown that cellular agriculture can have positive implications on animal health and welfare. The control that cellular agriculture offers over the quality of produced feed (i.e., GM/GE techniques that allow for specific proteins to be produced) can provide balanced nutrients for optimal animal growth and meat quality (e.g., reduced unnecessary fats) [Mattick 2018]. Moreover, cellular protein production typically takes place under hygienic conditions in controlled environments (e.g., lab-like facilities), therefore reducing the risk of potential biological contaminations (e.g., mycotoxins) that can threaten animal health. However, safety risks that include biological and chemical contamination may arise if the substrates (e.g., crop by-products) and facilities (e.g., fermentation tanks) are contaminated during production, or if the produce is contaminated at handling and post-production processing [Teng et al., 2021]. These risks become particularly relevant in the absence of technologies required for the monitoring and detection of undesirable microbial communities, toxins and chemical pollutants (e.g., nanoplastics), especially if waste substrates are used for production.
Finally, acceptability by livestock of such novel feeds can present an issue for animal welfare. For example, characteristics of novel feeds like palatability or texture compared to conventional protein feeds may cause livestock to consume less quantities and therefore also risk good animal health and growth [Mainardes & DeVries, 2016]. However, small adaptations in management practices (e.g., social feeding strategies, gradual adaptation) can help greatly increase the willingness of livestock to consume alternative feed ingredients [Mainardes & DeVries, 2016].
Social development
Several opportunities for social development may arise with the development of cellular agriculture markets. Novel protein production systems could be located adjacent to sources of waste and industrial by-products, therefore establishing efficient waste-to-product chains and greatly improving waste disposal [Williams, 2021]. Cellular protein production facilities that are located in urban areas can help build more resilient and resource-efficient localised livestock systems that do not rely on transportation of ingredients over long distances and contribute to the development of local economies. The requirements cellular agriculture has for specialised labour and renewable energy sources can further encourage social and economic growth of less developed rural communities.
Consumer perception and acceptance
Consumer acceptance is not considered a serious threat to the marketability and economic viability of cellular protein feeds, since cellular agriculture has become an established practice in the food industry over the past two decades. Consumers have gained significant exposure to foods arising from cellular agriculture processes, especially used in vegetarian and vegan diets, which should enable the smooth incorporation of such alternatives in livestock feeds [Teng et al., 2021; Zollman Thomas & Bryant, 2021].
Potential risks regarding the willingness of consumers to try livestock products reared with cellular protein may arise when waste substrates or GM/GE – bioengineering techniques are used that are not well-understood or trusted by sections of the public. Further educating consumers on the overall environmental, economic and social benefits of this alternative, on wrong perceptions of related food safety issues, and adhering to transparent labelling protocols can help enhance consumer acceptance and adoption rates.
Food Safety
As also discussed for animal health and welfare above, a major concern for food safety arises when cellular protein production uses waste substrates that have not been thoroughly processed. Such practices introduce the risk for several biological (incl. viral, bacterial, or fungal microorganisms) and chemical contaminants (incl. microplastics, heavy metals, synthetic molecules) to enter the human body through bioaccumulation. While current research is constantly uncovering new information, there is still much uncertainty around the precise mechanisms through which such contaminants are enabled and the specific problems they may cause to humans.
Another concern for food safety relates to that synthetic protein may lead to the introduction of allergens in the human food chain similar to conventional protein sources, therefore leading to potential allergic reactions and intolerances through the consumption of livestock products [Kuhad et al., 1997].
Regulatory implications
A strict regulatory system must be in place to address safety related issues particularly when waste substrates and GM/GE techniques are used for cellular protein production. Early and precise detection of biological and chemical contaminants, as well as monitoring of good hygiene practices are critical to ensuring feed and food safety. Anticipatory monitoring strategies, such as randomised sampling and thorough inspections of production chains, as well as strict labelling policies should be developed to prevent feed and food fraud incidents, which may increase as quality and affordability of cellular protein improve and the market expands.