The Future of Animal Feed: Drivers for shift from conventional protein ingredients to local, soilless and circular alternatives in livestock feeds
Livestock feed is the largest user of available agricultural land globally covering approximately two times more land than food crop production.
Environmental drivers
Livestock feed is the largest user of available agricultural land globally covering approximately two times more land than food crop production, with the increase in soybean cultivations being a primary driver for land-use change (Fig 1.-2.) [Manceron et al., 2014]. While technological advances and improved management practices in livestock production have driven a reduction in pasture land-use over the past two decades, global cropland for livestock feed has exhibited an increasing expansion particularly in the global South [Winkler et al., 2021]. The expansion of soy production for livestock feed has been associated with increased land degradation and land-use related impacts (i.e., deforestation) in one of the most important biodiversity hotspots globally, the Brazilian tropical savanna (Cerrado) (Fig.2) [Song et al., 2021]. While, strict policies have been implemented to mitigate severe environmental impacts of farming expansion, such as deforestation (e.g., the “Soybean Moratorium”) [Kastens et al., 2017], this had limited effect in reducing the intensity of resource use including land [Lathuilliere et al., 2017].
Figure 1: Expansion of the share of conventional protein crops in livestock feed formulations between 1961-2009 based on FAOSTAT. Source: Manceron et al. (2014)
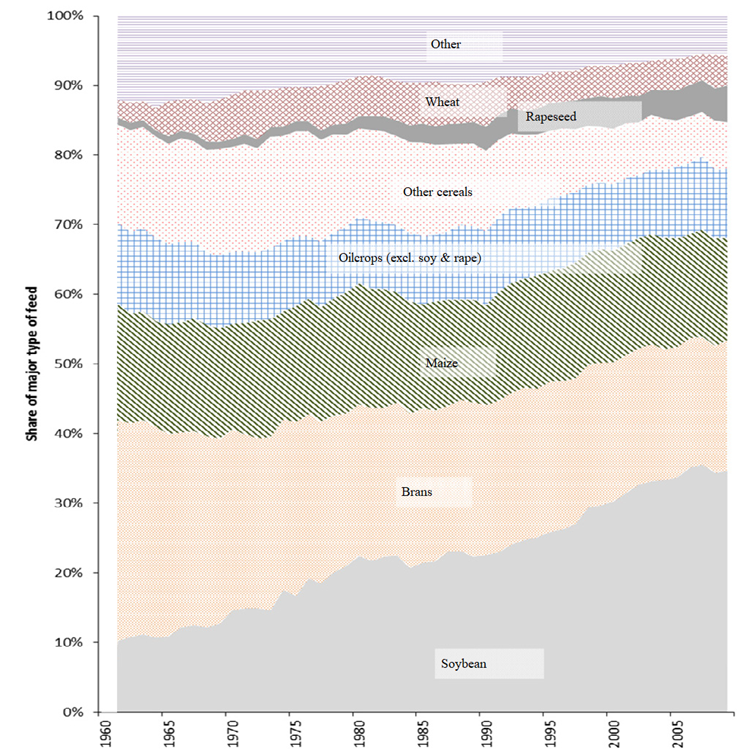
Figure 2: Land-use impacts by soybean crop expansion in South America presented through overlays of annual soybean classification maps from 2001 to 2019. Source: Song et al. (2021)
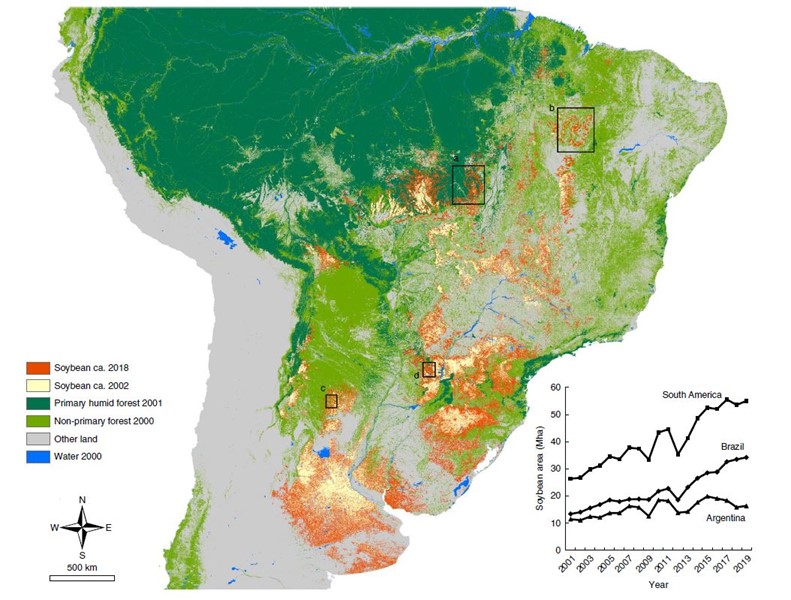
Many of the land-use related impacts discussed previously are also associated with greenhouse gas emissions (GHGs) arising from protein crop production [Castanheira & Freire, 2013]. Soil management practices affect the release of CO2 from the land and into the air, and therefore adopting alternatives that help reduce the need to disturb the soils can positively contribute in the reduction of anthropogenic GHGs [Johnson, 2018]. Furthermore, the expansion of the protein crop sector in the past two decades has led to a global increase in biomass burning, a practice used for deforestation, largely contributing to anthropogenic climate change and atmospheric pollution [Hoang & Kanemoto, 2021]. In addition to slash-and-burn deforestation, the burning of agricultural wasted biomass from conventional protein crops has worsened issues of smoke haze and airborne particulate matter pollution in the global South, particularly South East Asia and Brazil [Tang & Yap, 2020; Adam et al., 2021]. Therefore, shifting to more soilless and circular protein sources has an important positive interaction between mitigation of the land-related impacts discussed previously and the opportunity to greatly reduce global warming potential and atmospheric pollution associated with protein of livestock feed. Another source of GHGs from conventional crop production is associated with the main hotspots for energy and fossil fuel use, which are the operation of machinery for field operations (~63% of total energy-use) and the production of necessary synthetic fertilisers, herbicides and pesticides (~37% of total energy-use) [Hoffman et al., 2018].
Interlinked with the land-use related impacts mentioned previously, are also direct impacts to biodiversity. Disturbances of forest ecosystems (i.e., deforestation) by crop farming expansion, among other industries, is a big driver of species extinction of indigenous flora and fauna [Semper Pascual et al., 2019]. Together with restrictions enforced by several nations globally regarding the importation of crops that cause deforestation (e.g., UK Soy Manifesto https://uksoymanifesto.uk/faq/), soilless and circular alternative protein sources may present much needed opportunities towards biodiversity conservation, especially when they are implemented in regions that are considered as biodiversity hotspots e.g., Brazilian Cerrado [Lima et al., 2019; Paiva et al., 2020]. However, as previously discussed it is important that shifting to such solutions does not leave behind abandoned and degraded land. Degraded soils cannot support plant succession with a healthy organic horizon (i.e., humus) and sufficient nutrients and this can have detrimental effects on the diversity of plant species. A secondary effect of land degradation is habitat fragmentation (e.g., fragmented forest ecosystems), and so if conventional protein crops are replaced then the maintenance of patches that allow species and gene flow should be considered [Pacheco et al., 2018].
Furthermore, the use of large amounts of nitrogen and phosphorus synthetic (e.g., urea and di-ammonium phosphate) and organic (e.g., manure) fertilisers for conventional protein crop production is the primary contributor to acidification and eutrophication impacts associated with livestock feeds, both for the terrestrial and aquatic ecosystems [FAO, 2016a; Zortea, Maciel & Passuello, 2018].
Finally, agriculture is the largest contributor to water stress globally, with approximately 57% of the blue water footprint of global conventional crop production being unsustainable [Mekonnen & Hoekstra, 2020]. Oil crops (e.g., soybeans, rapeseed, sunflower) are the primary sources of protein in livestock diets requiring an average of 2400 m3 of water per ton of produce; the second highest crop class in water requirements, after pulses (e.g., beans, peas, lentils) [Mekonnen & Hoekstra, 2011].
Economic drivers
The cost of production and supply of conventional protein crops involves large financial investments associated with necessary direct inputs, including planting material (e.g., seeds), fertilisers and water for growth, and plant protection products (e.g., pesticides, herbicides). Major costs are also incurred due to labour inputs, fuel consumption for on-farm operations (e.g., tillage, spray runs), and for the land (e.g., rent) [FAO, 2016b]. Transporting large amounts of crops over long distances, often internationally and involving various means of transport (e.g., ships, planes) is another source of significant costs. In the case of soybean that comes from Brazil, which is a major global supplier, there are additional, high logistics costs due to poor infrastructure connecting the producing region to the exporting hubs, even when alternative routes are explored [Oliveira et al., 2020]. Furthermore, due to the dependency of conventional protein crop production on fossil fuel (i.e., oil, petrol), the volatility of the price of such inputs can often cause price spikes and interruptions in the supply of critical livestock protein feeds, such as soy and sunflower meals [Taghizadeh-Hesary, Rasoulinezhad & Yoshino, 2019].
Food safety and social drivers
The conventional feed production and processing system exposes protein ingredients to several biological and chemical contaminations at high rates and throughout the various phases, such as pesticides at production and fungal contaminations at storing and transportation. One particularly concerning type of such contaminations is caused by mycotoxins. These can have carcinogenic, immunosuppressive, mutagenic and teratogenic implications for animal and human health [Kabede et al., 2020]. Aflatoxin, a naturally occurring mycotoxin produced by the Aspergillus flavus fungus, is known as the most potent hepatocarcinogenic molecule in nature and can cause stunted growth, breathing difficulties, hormonal imbalances, reproductive diseases and even lead to death [Conte et al., 2020; Mahato et al., 2021]. Oilseeds including soybeans, rapeseed and sunflower seeds that are subjected to stressful conditions like prolonged heat, drought, damages by insects, and damages or moisture at transportation/storage are vulnerable to A. flavus contamination, which appears as yellow-green or gray-green mold on the seeds. If these seeds are then used as livestock feed, they pose a serious threat to animal health due to increased levels of the aflatoxin. Through livestock products such as meat, milk and eggs, humans then get exposed to aflatoxins. These contaminants are persistent and can be found even in human milk through the form of other metabolites, threatening infants’ healthy development [Alshannaq et al., 2018]. Therefore, it is important to explore solutions that allow for more resilient genotypes and shorter production and supply chains to avoid contamination risks. Furthermore, diversifying crop production with the adoption of alternative protein feeds may present further opportunities for social development as it would increase the overall size and economic value of the livestock feed production sector (currently 62% of the European cereal crops, 88% of soy, and 53% of pulses used as animal feeds) (FAOSTAT, 2021).
Local, soilless and circular alternatives as a potential solution
A wealth of research has focused on the nutritional value, digestibility, and use of alternative protein feeds for livestock. Literature suggests that alternative protein from local, soilless and circular sources can sufficiently substitute conventional feed ingredients from a nutritional perspective, although soy is still considered among the most balanced protein feeds for optimal animal growth [Gasco et al., 2019; Luciano et al., 2020]. In addition, such alternative protein sources may present solutions to address the critical sustainability issues of conventional feed production discussed above. In the following chapters, this report presents opportunities and risks associated with the incorporation of alternative protein feed ingredients sourced from:
- Genetically modified / engineered (GM/GE) protein crops and alternative protein crop growing methods (Chapter 3)
- Cellular agriculture (Chapter 4)
- Former foods, food waste, industry by-products and waste streams (Chapter 5)
- Animal by-products and insects (Chapter 6)
The classification above is in accordance with practices from recent scientific literature and was selected following consultations with experts from the FSA and the Department for Environment, Food & Rural Affairs (DEFRA). The first group represents plant-derived protein from alternative crop types (e.g., GM/GE variants, legumes) or alternative plant growing methods (e.g., hydroponics). The second group encompasses practices for mass protein extraction at a microscopic level (e.g., fungal, bacterial, micro-algal organisms). The third group represents circular agriculture streams using waste and by-products of the agricultural and other industries (e.g., brewing, bio-refinery). Finally, the fourth group includes processed animal proteins (PAPs) from by-products of livestock production or from insect farming.