The Future of Animal Feed: Former foods, food waste and industry by-products and waste streams
This sections explains the foods, food waste and industry by-products and waste streams from animal feed focusing on; environmental, economic and social implications.
Supplying livestock directly with human inedible protein from food waste has been another major topic of research as a potential solution to livestock feed sustainability without compromising food availability, since approximately 30% of global arable land produces food that is subsequently not eaten [FAO, 2013; Tonini et al., 2018].
The assessment distinguishes between food waste and former foods. The former, refers to edible material that was discarded either by choice or due to events like spillage and spoilage during production and processing, while the latter refers to foods or food ingredients that did not reach retailers and consumers (e.g., due to not meeting quality criteria). It is important to consider that food waste may contain some inedible material mainly due to packaging residues [Tavill, 2020].
Many studies have investigated the nutrient profiles of popular food wastes globally, the impact of waste processing methods on the effectiveness of food wastes as feed, and the potential implications on livestock performance across several species [Dou, Toth & Westendorf et al., 2018; Luciano et al., 2020]. Evidence suggests that food waste from both hospitality and households have potential to substitute protein feedstuffs, while former foods from bakery and confectionery can be used as a source of protein in livestock diets [Pinotti et al., 2021; Rajeh et al., 2021]. Many large European and UK retailers, including Tesco, Arla Foods, and Coca Cola, have already started investing in their waste sorting procedures to safely use surplus food as animal feed [WRAP, 2016]. Such actions however have been delayed by significant food security threats, including the Covid-19 pandemic, that forced retailers, restaurants, hotels and cafeterias to operate at very low capacity, therefore generating limited amounts of food waste.
Crop residues, agro-industrial by-products, and agroforestry plants (e.g., tea, cassava, mulberry, mesquite) also present opportunities to source proteins for use in livestock feed. These sources refer to by-products that are inedible for humans, such as plant foliage and by-products (e.g., leaf protein concentrates) derived from agroforestry and post-harvest crop processing and that can be used as sources of protein, energy and fibre for livestock [Tallentire et al., 2018; Li et al., 2019; Salami et al., 2019]. Distillers’ dried grains with solubles (DDGS), a by-product of the biofuel industry, has been among the most popular examples used to substitute soya and maize in diet formulations for livestock and fish [Makkar, 2018]. Due to the potential use of such by-products as livestock feed, synergies and integrations between biorefineries and livestock systems have been explored to address issues of land availability and redistribute the environmental pressures associated with the two industries [de Souza, Junqueira & Cavalett, 2021].
Agri-food waste-streams present many opportunities to sourcing protein for livestock feed, while at the same time addressing a range of environmental pollution issues. Using novel biotechnologies, nitrogen recovery rates of close to 100% have been reported from waste and wastewater through to microbial protein for cellular protein, revealing the potential for biobased circular economies in agriculture [Puyol et al., 2017]. Recent research has also explored the potential of waste from livestock (e.g., liquid slurries) and biorefineries (e.g., anaerobic digestate) to help grow sustainable duckweed, minimising water use for its cultivation and land otherwise used for the disposal of the excess slurry [Stadtlander et al., 2019; Sońta et al., 2020].
Environmental implications
Land degradation, land use change and land availability related impacts
Generating protein from former foods, food waste, and industry by-products can help mitigate global livestock sector land-use footprint, as it alleviates pressures from land that would otherwise be used for feed-crop production. Funnelling food and industry wastes into livestock feeds can significantly reduce requirements for landfills and relieve overall pressures on waste disposal systems [Tonini et al., 2018]. Land occupied by plant, cereal and fruit production in particular, can be used to accommodate grazing needs by ruminants, in addition to reducing crop land requirements [Schader et al., 2015; Salami et al., 2019].
Greenhouse gas emissions, atmospheric pollution and fossil fuel depletion
Treating waste streams as valuable by-products helps significantly reduce the overall environmental footprint of the industries that generate them – i.e., bakery, hotels and restaurants, crop production, brewery, biofuel, etc. This is because the allocation of emissions and pollutants generated through production, including GHG atmospheric emissions and impacts to resource availability, would change from an “only the primary product is responsible” allocation process, to “emissions are allocated to the primary product and valuable co-products”. Incorporating such soilless protein sources in livestock feed frees up land and resources for conventional protein crops like soybean, which can be used directly for human consumption or by other industries (e.g., as feedstock for biofuel), overall reducing the environmental footprint of livestock feed production. Studies have shown that using food waste as feed is the best performing disposal strategy from an environmental perspective, when compared to the use of food waste as feedstock for anaerobic digestion (i.e., bioenergy) and composting (i.e., organic fertiliser) (Fig.4) [Vandermeersch et al., 2014; Salemdeeb et al., 2017; Dou, Toth & Westendorf et al., 2018].
With these alternatives, it is important that any required pre-treatment methods for the different potential uses for such waste-streams are considered as their environmental impacts and resource requirements may vary significantly (Fig. 5).
Figure 4: Environmental burdens and benefits of different uses for food waste – as dry (grey) and wet feed (brown) for livestock production, through anaerobic digestion (blue), and composting (green). The following environmental impact categories are considered: GWP = Global Warming Potential, ODP = Ozone Depletion, HT-C = Carcinogen Emissions, HT-NC = Non-carcinogenic Toxin Emissions, IR = Ionising Radiation, POF = Photochemical Oxidant Formation, FEP = Freshwater Eutrophication Potential, MEP = Marine Eutrophication Potential, ET = Ecotoxicity, ADP-F = Fossil Fuel Depletion, ADP-E = Abiotic Resource Depletion, AP = Acidification Potential, TEP = Terrestrial Eutrophication Potential, PM = Particulate Matter Emissions. Source: Salemdeeb et al. (2017)
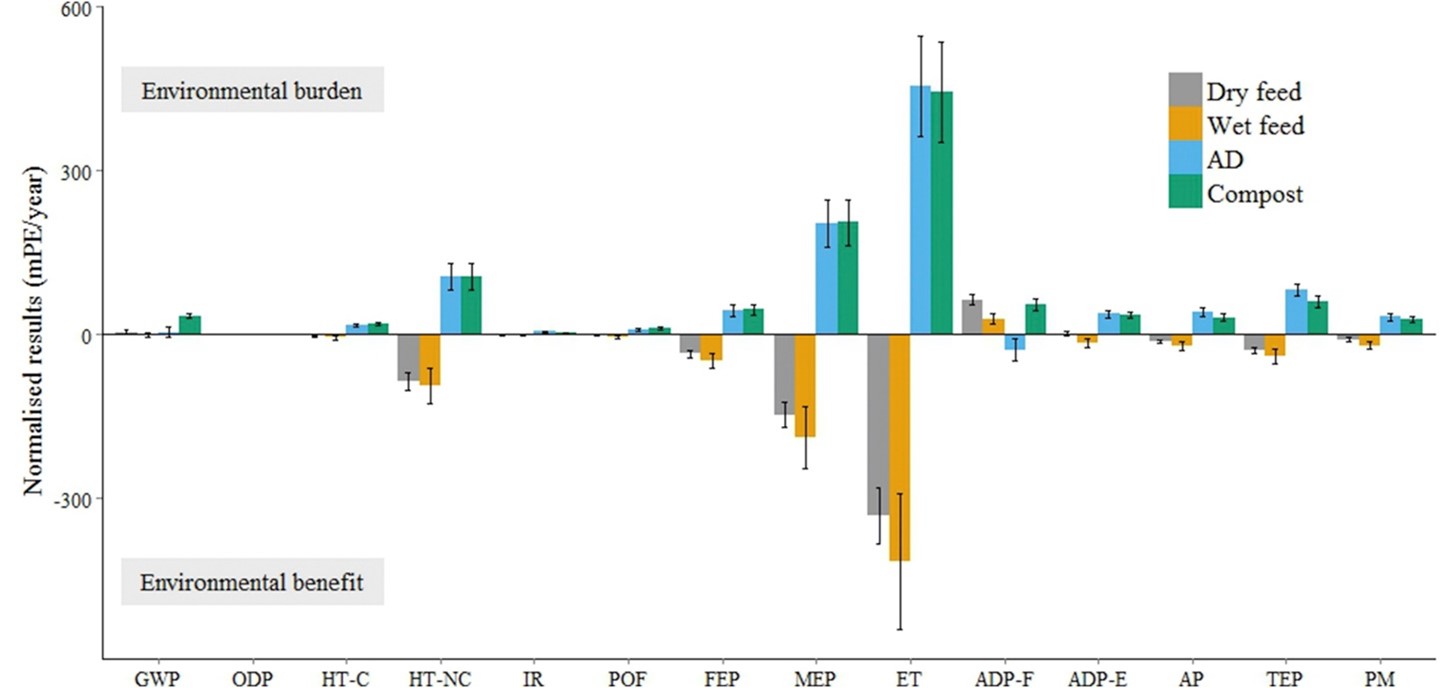
Figure 5: Fossil fuel requirements for the production of dry and wet feeds from food waste. The figure highlights the importance to consider relevant treatment and processing requirements associated with different disposal strategies for food waste, even when comparing different animal feeding strategies. Source: Salemdeeb et al. (2017).
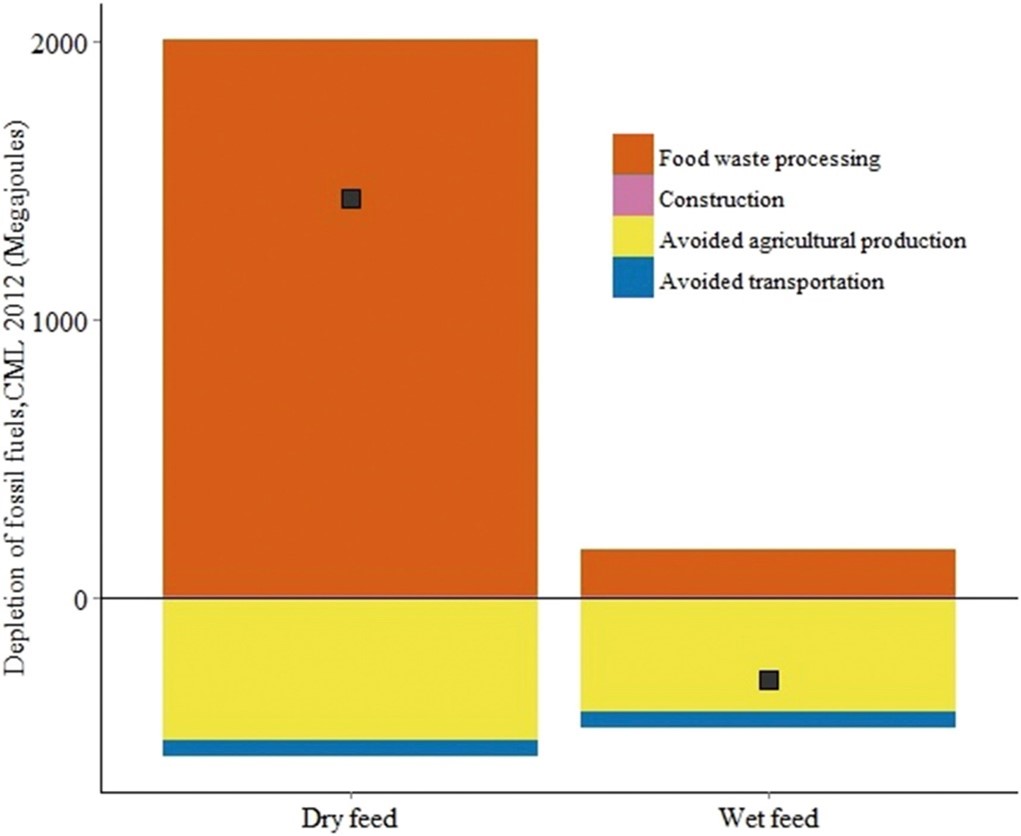
Nitrogen and phosphorus related impacts
Utilising former foods and food waste to source protein creates opportunities towards reducing acidification and eutrophication impacts, even when compared to other potential exploitation avenues (e.g., anaerobic digestion for energy production, composting as organic fertiliser) (Fig. 2) [Salemdeeb et al., 2017]. Production of feed from food waste is not dependent on synthetic fertilisers and other chemical inputs, therefore reducing pressures on fresh and marine water bodies from nutrient and chemical leaching. Furthermore, it enables a recycling path for nutrients that would otherwise be deposited to soils and aquatic ecosystems through waste disposal.
Impacts to water quality and depletion of water resources
Large improvements in water efficiency of livestock feeds can be achieved through sourcing of protein from food waste and industrial waste streams, while at the same time and through the same process removing unwanted contaminants from fresh and marine water bodies [Dou, Toth & Westendorf et al., 2018]. Substituting conventional feeds with biofuel, agroforestry, and plant by-products may help reduce the water footprint of these production chains overall, provided that increased demand of such co-products does not drive overall increases in production [Mekonnen & Hoekstra, 2020].
Impacts to biodiversity
The biodiversity impacts related to the disposal of food waste are far smaller than the ones related to the production of food that is eventually wasted [FAO, 2013]. Therefore, using food waste as feed yields its greater benefits for biodiversity conservation indirectly, sharing the environmental burden associated with food production as a valuable co-product (i.e., re-allocation of environmental impacts). Direct, positive impacts on biodiversity result from the fact that converting food waste to feed does not require any additional land or synthetic and chemical inputs compared to conventional agriculture, which helps preserve good habitat quality and reduces habitat fragmentation.
Economic implications
Production and supply economics and resilience to extreme events
Circular agriculture streams for protein sourcing, such as feed derived from food waste and former foods, may also help the livestock sector avoid large costs associated with conventional protein crop production. A critical condition for these alternatives to be viable and cost-effective is the proper treatment of food waste prior to their use as feed, to reduce the risks of pathogen and disease outbreaks that could lead to severe economic consequences for the livestock sector [Dou, Toth & Westendorf et al., 2018]. Ensuring high hygiene standards through timely collection of the waste, thorough thermal treatment, appropriate transportation and handling practices incurs costs that need to be accounted for when evaluating the feasibility of such feeding strategies [Pinotti et al, 2020; Rajeh et al., 2021]. When comparing potential uses of food waste, specifically processing it for the production of energy (i.e., anaerobic digestion) or processing it for the production of animal feed (i.e., hygienic / thermal processing), the latter incurs twice the cost [Martinez-Sanchez et al., 2016]. However, the financial benefits the livestock sector gains by reducing animal feed production this way, generally outweigh the costs and make it a potentially viable alternative [Martinez-Sanchez et al., 2016; Pinotti et al., 2021]. Further advancements in biotechnology for waste treatment may increase the cost-effectiveness of circular agriculture alternatives (e.g., protein from food waste, insects reared on waste) and cellular agriculture protein streams (e.g., bacterial protein produced from waste substrates), thereby enabling easier implementation at large scales [Ritala et al., 2017; Jones et al., 2020].
Social implications
Nutritional value and animal growth
Food waste can be a good source of amino acids, minerals, fatty acids, and vitamins essential for animal growth [Rajeh et al., 2021]. However there is no clear evidence that it can significantly improve animal performance. Literature suggests that food waste does not affect meat quality, while some studies propose it may even improve it [Dou et al., 2018]. Table 1 below as adapted from Rajeh et al. (2021), summarises the potential for food waste streams to provide valuable nutrients for animal growth when incorporated in livestock feeds.
Table 1: Nutritional value of various sources of food waste used as livestock feeds and tested on various livestock species. Adapted from Rajeh et al. (2021).
Food waste | Tested livestock | Dry matter % | Crude protein % | Crude fibre % | Optimal inclusion level (% of total feed) | Feed conversion ratio (kg intake/kg weight gain) |
---|---|---|---|---|---|---|
Retail food waste | Fish | 97.9 | 19.6 | - | 20 | 1.5 |
Restaurant food waste | Fish | 91.1-97.9 | 18.9 - 31.1 | 0.8 - 15.3 | 25 - 75 | 1.1 - 1.3 |
Hotel food waste | Fish | 93.2-97.9 | 19.6 - 31.1 | 5.7 | 20 - 53 | 1.5 - 2.6 |
Pasta waste | Fish | 89.8-91.5 | 12.7 - 14.3 | 0.3 - 2.0 | 30 - 75 | 0.9 - 2.5 |
Date waste | Fish | 86.7-92.9 | 2.4 - 2.6 | 1.8 - 2.1 | 30 | 1.2 - 1.8 |
Biscuit waste | Chickens | 89.3-92.0 | 5.3 - 12.6 | 1.1 - 2.6 | 24 - 50 | 1.9 - 2.5 |
Restaurant food waste | Chickens | 82.1-93.7 | 15.8 - 16.0 | 2.0 - 8.9 | 10 - 20 | 3.3 - 4.6 |
Retail food waste | Chickens | 82.1 | 15.8 | 2.0 | 20 | 4.6 |
Kitchen (household) food waste | Chickens | 87.6 | 15.8 | 10.8 | 5 | 3.9 |
Fruit waste | Chickens | - | 6.9 | - | 12.6 | 2.0 |
Date waste | Chickens | 95.2 | 8.1 | 9.1 | 10 | 2.3 |
Noodle waste | Chickens | 94.7 | 8.8 | 1.5 | 50 | 1.9 |
Retail food waste | Cows | 94.5 | 20.0 | - | 25 | - |
Kitchen (household) food waste | Cows | 85.3 | 20.1 | 9.7 | 50 | 7.3 |
Cafeteria food waste | Cows | 46.1 | 29.4 | - | 50 | - |
Vegetable waste | Goats | 28.7 | 8.3 | - | 82 | 33.5 |
Restaurant food waste | Pigs | 19.1-96.1 | 14.4 - 25.0 | 2.3 14.5 | 20 - 50 | 3.0 - 5.6 |
Fruit waste | Pigs | 93.6 | 19.5 | 6.2 | 4.0 | 3.2 |
Kitchen (household) food waste | Pigs | 23.1 | 14.1 - 19.1 | 4.2 - 4.4 | - | 3.0 - 3.1 |
Bakery waste | Pigs | - | 9.5 | 2.3 | 24 | 3.2 |
Biscuit waste | Pigs | 84.5 | 8.7 | 0.3 | - | 3.2 |
Cafeteria food waste | Pigs | 22.4-89.7 | 17.8-21.4 | - | 20 - 50 | 3.4 - 4.3 |
Retail food waste | Pigs | 89.7 | 17.8 | - | 20 | 4.3 |
Restaurant food waste | Sheep | 89.5 | 14.7 | - | 15 | 6.9 |
Kitchen (household) food waste | Sheep | 89.5 | 14.7 | - | 15 | 6.9 |
Biscuit waste | Sheep | 96.9 | 9.7 | 2.1 | 25 | 5.0 |
Animal health and welfare
Food waste and former foods need to be very carefully treated with thermal processing (e.g., hydrothermal treatment at 110 °C for an hour) before they are fed to animals, because they can contain viruses and bacteria that may cause serious animal diseases like swine fever and foot-and-mouth disease. Even when food waste, former foods and industry waste streams are safe from a microbiological perspective (e.g., from microbial and fungi contaminants) they may contain high levels of chemical residues from packaging (e.g., plastic) and processing (e.g., heavy metals, pesticides) causing harm to farmed animals [Pinotti et al., 2019]. Some former foods and food waste may contain high concentrations of secondary metabolites or toxins, for example chocolate residues can be high in theobromine. For this reason, their levels of inclusion in livestock feeds need to be carefully considered.
While literature suggests that packaging remnants in former foods and food waste are usually negligible (i.e., less than 0.1g per 100g), it is important to consider that different types of materials may exhibit very different resistances. For example, polyethylene and polypropylene which are two common packaging plastics, have different melting points at ~120°C and ~240°C respectively.
Social development
Upcycling of food wastes and waste streams through livestock feeds creates several opportunities for social development boosting growth of local communities, driving innovation in biotechnologies and creating jobs for specialised labour [European Commission, 2015]. Adopting circular alternatives helps improve ecosystem services, enhance quality of ecosystems, conserve habitats for wildlife, and reduce pressures from agricultural intensification for conventional crop production, which improve overall quality of life particularly for rural communities [Popescu, 2019; Zhu et al., 2019; Jagtap et al., 2021].
Consumer perception and acceptance
Evidence suggests that consumers of livestock products are currently conflicted with regards to the suitability and safety as livestock feeds of food wastes, former foods and industry waste streams, and insects reared on such waste streams. On one hand, studies have shown that consumers understand the benefits and appreciate the feeling of “doing good” by generating valuable nutrients while reducing waste and improving the environmental and economic performance of global livestock systems (i.e., “Eco-feed” concept) [Saito et al., 2009; Borrello et al., 2017; Bhatt et al., 2018]. On the other hand, the possibility that food waste can be contaminated by pathogens that caused recent outbreaks of diseases like Covid-19 and African Swine Fever, raises issues of distrust regarding hygiene standards in livestock facilities, and discouragement among livestock producers and consumers from supporting adoption of this circular alternative [Mens et al., 2021; Jayathilake et al., 2022].
Food safety
As also discussed in previous chapters, microplastics found in food wastes can reach humans bodies posing serious threats such as genotoxicity, inflammations and cell apoptosis. Besides the pathway for human exposure to microplastics through ingestion of contaminated livestock products, studies have identified another indirect and even more potent route. Specifically, research has shown that digested microplastic, i.e., microplastic that has been fed to livestock through food waste feeds and then excreted after digestion, has altered properties that increase its adsorption capacity. This means that once released in wastewaters or waste substrates through manure disposal it can be available as accidental feed for a wider group of organisms (e.g., fish, insects) and eventually reach humans through several bioaccumulation pathways [Krasucka et al., 2022].
An important risk for food security arises with the potential for several allergens to reach human foods through the use of food waste as animal protein feeds. It is important to consider that waste from restaurants, households, retailers, cafeterias, and bakery and confectionery frequently contain the majority of the 14 major allergens – gluten, dairy, fish and egg proteins, and traces of nuts, groundnuts and peanuts [ACAF, 2009; Testa et al., 2017; Bingemann et al., 2019]. Aside from allergens, food waste can contain various additives and processed ingredients, such as artificial sweeteners (e.g., aspartame), flavour enhancers (e.g., monosodium glutamate), artificial colour additives and others, which can reach humans through bioaccumulation in livestock tissues. These chemical substances have been associated with harmful effects on humans including respiratory and skin allergic reactions and even stomach and intestinal cancer [Gultekin et al., 2020; Rinninella et al., 2020].
Regulatory implications
The safe adoption of food wastes and waste streams as animal feeds presents a great challenge for regulatory systems of the global livestock sector. Similar to the case of cellular agriculture, it is critical that safety assessment protocols use state-of-the-art biotechnologies to detect biological and chemical contaminants in food waste early and with precision. There is a need for sensitive detection and treatment methods to ensure safe implementation of such alternatives, as studies have reported concentrations of heavy metals and pesticides in food waste that exceed limits allowed in conventional protein sources [Dou et al., 2018]. Establishing standards and schemes for the certification of treatment techniques and practices, and types of wastes that are appropriate for conversion to livestock feed may be required to avoid relevant food safety threats [Westendorf,, 2000]. Unmonitored and unregulated processing and feeding practices can lead to large disease outbreaks with severe consequences for the growth of the livestock sector, like the 2001 Foot-and-Mouth disease in the United Kingdom [Mens et al., 2021]. Such incidents further contribute to feelings of distrust by the consumers and lack of confidence in that regulatory authorities can guarantee the safety of novel feeds and consequently of livestock products.